An Industrial Perspective on Magnesium Alloy Wheels: A Process and Material Design
Miaomiao Wang1,2
1China Copper Institute of Engineering and Technology, Beijing, China.
2Kunming Metallurgical Research Institute Co., Ltd. Beijing Branch, Beijing, China.
DOI: 10.4236/msa.2023.141002PDFHTML XML18 Downloads 134 Views
Abstract
Light weights wheels improve vehicle performance with respect to road handling, cornering as well providing fuel economy and reduced greenhouse gas emissions. Aluminum wheels are currently used in many models and are produced usually by low pressure assisted gravity casting. Important property requirements are fatigue strength, pressure tightness, tensile strength, impact resistance, and corrosion resistance. Many attempts have been made to convert aluminum road wheels to magnesium. Race cars and some of the high end models (Porsche, Ferrari, etc.) have used magnesium wheels. These wheels have been gravity cast or forged. Viable corrosion protection systems have been developed and magnesium wheels have been used with success on these models. To use magnesium on more modest models is a challenge due to cost issues. Higher productivity casting processes or more cost effective coating systems need to be utilized. The project consists of selecting magnesium alloys for road wheels, examining the possible cost effective casting processes and corrosion protection systems, evaluating the cost per one wheel and comparing it to aluminum wheel costs. The wheels will also be compared with respect to fatigue and impact properties, pressure tightness, and corrosion.
Abstract Korea
경량 휠은 도로 핸들링, 코너링과 관련하여 차량 성능을 향상시키고 연비를 제공하고 온실 가스 배출을 줄입니다. 알루미늄 휠은 현재 많은 모델에 사용되며 일반적으로 저압 보조 중력 주조로 생산됩니다. 중요한 특성 요구 사항은 피로 강도, 압력 기밀성, 인장 강도, 내 충격성 및 내식성입니다. 알루미늄로드 휠을 마그네슘으로 변환하려는 많은 시도가있었습니다. 경주 용 자동차와 일부 고급 모델 (포르쉐, 페라리 등)은 마그네슘 휠을 사용했습니다. 이 바퀴는 중력 주조 또는 단조되었습니다. 실행 가능한 부식 방지 시스템이 개발되었으며 마그네슘 휠이 이러한 모델에서 성공적으로 사용되었습니다. 보다 겸손한 모델에 마그네슘을 사용하는 것은 비용 문제로 인해 어려운 일입니다. 더 높은 생산성의 주조 공정 또는 보다 비용 효율적인 코팅 시스템을 활용해야 합니다. 이 프로젝트는 로드 휠용 마그네슘 합금을 선택하고, 가능한 비용 효율적인 주조 공정 및 부식 방지 시스템을 조사하고, 한 휠당 비용을 평가하고, 알루미늄 휠 비용과 비교하는 것으로 구성됩니다. 휠은 또한 피로 및 충격 특성, 압력 기밀성 및 부식과 관련하여 비교됩니다.
Keywords
Manufacturing Process, Die-Casting, Corrosion Resistance, Economic Viability
Share and Cite:
Wang, M. (2023) An Industrial Perspective on Magnesium Alloy Wheels: A Process and Material Design. Materials Sciences and Applications, 14, 20-44. doi: 10.4236/msa.2023.141002.
During the most part of 20th century, magnesium alloy developments have traditionally been driven by aerospace industry requirements for lightweight materials to operate under increasingly demanding conditions [1]. There are two important reasons for this development. The first reason is that during the 1990’s, there was an increasing environmental awareness with the aim of conserving resources and reducing harmful emissions. Another reason is that magnesium alloys have always been attractive to designers due to their low density, only two thirds that of aluminum (Al) [2]. In both cases, the objective is to reduce fuel consumptions and the result has been a rediscovery of magnesium as a lightweight construction material for automotive wheels industry. Recently, an additional requirement in magnesium alloys has been the superior corrosion performance. Due to the advances in mechanical properties and corrosion resistance, magnesium alloys have slowly gained their place in the aerospace industries [1].
With the help of modern innovation, magnesium alloy wheels have become increasingly popular in the automotive industry, to meet the rising performance requirements by the automobile manufacturers. Reasons justifying auto makers to choose magnesium wheels are primarily focused on weight savings considerations. Magnesium alloy wheels, or mag wheels, are often used on racing cars in place of heavier steel or aluminum wheels.
In road wheels applications, magnesium is possible to offer a weight reduction of approximately 25% compared with aluminum wheels. The atomic density of magnesium is 30% less than aluminum; however, a larger volume of magnesium metal is required to obtain appropriate wheel design, reducing the weight savings to 25% [3]. Typically, the mass of one magnesium alloy wheel is approximately between 8 kg and 12 kg, depending on the model [8]. As a result, the use of magnesium alloy wheels by the original equipment manufacturers (OEMs) and by the aftermarket (AM) manufacturers is expected to gradually and steadily increase.
In one hand, numerous advantages of magnesium alloy wheels exist. For instance, with a density of 1.8 g/cm3, magnesium is the lightest of all structural metallic materials. Another advantage of magnesium wheels relies on their good dimensional stability and rigidity [4]. Note that the rigidity of a wheel is an important characteristic necessary to maintain correct kinematics of a motor vehicle’s suspension and steering [3]. On the other hand, the disadvantages of magnesium alloy wheels rely on the fact that they are flammable and have been banned in some forms of motorsport in the UK following fires which are extremely difficult to extinguish. In addition, magnesium alloy wheels have been known to catch fire in competition use after a punctured tire has allowed prolonged scraping of a wheel on the road surface [5]. Another disadvantage is that some variants of magnesium alloy wheels may have low corrosion resistance. Moreover, most magnesium alloy wheels are expensive and are not practical for most street vehicles. Currently, most of them are being used in the high-end sports cars or luxury cars. For example, Porsche AG uses the magnesium alloy AZ91 wheels (7.44 kg each) with its 944 turbo model, and Honda uses the magnesium alloy AM60 wheels (5.90 kg each) with its Prelude model.
The nomenclature used to designate magnesium alloys is assigned by the ASTM (American Society for Testing and Materials). It gives an immediate, approximate idea of the chemical composition of an alloy, with letters representing the main constituents, and numbers representing the percentages of these constituents. The letters indicate the two principal alloying elements, arranged in order of decreasing percentage, (or alphabetically if percentages of the elements are equal). For example, aluminum-zinc-magnesium alloy AZ91 has the following designations: A represents aluminum, the alloying element specified in the greatest amount, Z represents zinc, the alloying element specified in the second greatest amount, 9 indicates the rounded mean aluminum percentage (between 8.6 and 9.4), and 1 indicates the rounded mean zinc percentage [6].
2. Magnesium versus Alternatives
2.1. Advantages and Disadvantages of Magnesium Use in the Automotive Wheels Industry and Comparison with Aluminum and Steel
Vehicle weight reduction plays an important role in improving automotive fuel efficiency [7]. Steel and aluminum wheels are already being used to reduce weight significantly, but additional reductions could be achieved with magnesium alloy wheels [8]. In fact, various magnesium alloys are already being used in relatively small quantities for automobile parts, especially in wheels [1].
Being the eighth most commonly found element on earth, magnesium is considered to be extremely abundant. Its main source of supply is seawater, which in turn contains 0.13% magnesium. Also, magnesium is a recyclable metal, and instituting a recycling system would extend supplies and save energy. Major increases in automobile usage would eventually require a production capacity expansion of magnesium [1].
As mentioned in Section 2, magnesium is an interesting material for the production of automotive wheels, mainly because of its light weight: it is 36% lighter per unit volume than aluminum and 78% lighter than iron (Fe). When alloyed with different metals, magnesium can achieve the highest strength-to-weight ratio of all metals. In addition, magnesium offers a great potential for weight reduction. Current production-model cars also contain various small magnesium castings, averaging around 2.72 kg/car. In the near future, increasing use of magnesium in the automotive industry is highly probable [1].
The use of magnesium alloy wheels is not just about their light weight—it is also about their moment of inertia. Although magnesium alloy wheels are the lightest wheels around, the most critical criterion of any wheel is its moment of inertia, and this is where magnesium wheel excels. Generally speaking, the moment of inertia increases with weight as a square function on a wheel from the center [9]; therefore, the lighter the wheel/tire combination is from the axle, the greater the improvement. Magnesium wheels have a relatively lower moment of inertia compared to steel or aluminum wheels. Also, magnesium wheels are up to 30 percent lighter than most of today’s advanced wheels, and even more significantly lighter than the standard production wheels [10]. Due to the fact that the weight saving comes mostly from the spokes and the rim, as a result, the mass of the wheel is mostly concentrated in the hub to the benefit of rotational inertia [2]. The latter affects the moment of inertia, which in turn is the critical factor that produces real benefits and greatly improves every aspect of an automobile’s performance, including turn-in, acceleration, and braking being most prevalent [9].
There are several limitations in using magnesium in the automotive industry. These limitations are mostly related to the physical properties of the metal [8]. Table 1 below compares the physical properties of magnesium, aluminum, and iron. It is important to note that magnesium is a relatively reactive metal. To overcome this problem, one may apply protective coatings on the metal, or allow the build-up of a naturally occurring oxide or sulfate coating. Although corrosion of magnesium has been a concern, nevertheless, due to the development of new magnesium alloys, such problem has been solved [4].
One of the main factors that prevent the massive use of magnesium is its relatively high and unstable price (the price of magnesium on January 1st, 2021 was between CAD$4295 to CAD$4500 per metric ton) [11]. On a per-pound basis, magnesium costs 3.5 to 6 times more than steel and 1.7 to 2.8 times more than aluminum. On a volume basis, the differential is considerably reduced, with
Properties | Magnesium | Aluminum | Iron |
Crystal Structure | HCP | FCC | BCC |
Standard Atomic Weight (g/mol) | 24.31 | 26.98 | 55.85 |
Density at 25˚C (g/cm3) | 1.738 | 2.70 | 7.87 |
Liquid Density at Melting Point (g/cm3) | 1.584 | 2.375 | 6.98 |
Melting Point (˚C) | 650 | 660 | 1536 |
Boiling Point (˚C) | 1091 | 2519 | 2862 |
Heat of Fusion (kJ/mol) | 8.48 | 10.71 | 13.81 |
Heat of Vaporization (kJ/mol) | 128 | 294 | 340 |
Heat Capacity at 25˚C (J/mol * K) | 24.869 | 24.2 | 25.1 |
Thermal Conductivity at 300 K (W/m * K) | 156 | 237 | 80.4 |
Thermal Expansion at 25˚C (µm/m * K) | 24.8 | 23.1 | 11.8 |
Young’s Modulus (GPa) | 45 | 70 | 211 |
Shear Modulus (GPa) | 17 | 26 | 82 |
Poisson Ratio | 0.29 | 0.35 | 0.29 |
Brinell Hardness (MPa) | 260 | 245 | 490 |
Table 1. Material properties comparison [2] [4] [9] [10].
magnesium’s price varying from 10% to 80% above that of aluminum and from 20% below to 30% above that of steel. It is also interesting to note that the joining or fabrication costs of magnesium may be lower compared to other metals, namely aluminum and steel. In fact, it is possible to have secondary weight savings (i.e.: of a car) by replacing heavy materials with lighter ones. Consequently, lifetime fuel costs are reduced. Thus, it can be said that the total life-cycle cost of a magnesium part may actually be lower than that of one made from another material [4].
Another important thing to consider is the fact that it is fairly easy to join magnesium parts. In the case of steel, the joining may require several steps; however, for magnesium it may only require one single step. Note that since magnesium is present mostly in the form of crystalline structures, its formation must be done at high temperatures (2000˚C to 3150˚C) [3]. Thus, it may be expensive to produce large part with magnesium.
2.2. Aluminum Losing Traction in Wheel Market
According to the “Demand and Supply Forecast for Metal Castings in the United States” by Stratecasts in 2012, manufactured steel wheels composed of about 15% of the automotive steel market, low pressure die-cast aluminum claimed approximately 75% of that same market, with the next closest cast metal—magnesium—at 5%. The remaining 5% of the market was held by other metals (i.e.: metal matrix composites). Stratecasts also forecasts that magnesium will make gains in the coming years, reaching 8% by 2020 [12].
Numerous magnesium alloys are available on today’s market. In order to choose the best alloy suitable for massive road wheels production, one must carefully study the alloy properties. Table 2 shows a list of potential magnesium alloys that are suitable for production. The latter alloys are compared with the minimum mechanical properties required for wheels production. Then, a first round elimination can be made based solely on the mechanical properties requirements for road wheels. After the first round elimination, 5 candidates have been selected: AZ31, AZ91, ZK60, AM20, and WE43. Subsequently, a second round elimination can be done based on the cost of these magnesium alloys and on their potential manufacturing process. Table 3 shows the cost as well as other characteristics of the remaining candidates. Based on Table 3, magnesium alloy WE43 has been chosen for the mass production of road wheels. First, only castable alloys can be selected due to the manufacturing process selected (refer to Section 4). Second, WE43 is the least expensive alloy among the other 2 castable choices (alloys supplier: Chang Chun Zhong Ke Xi Mei Magnesium Co. Ltd. in China). And third, WE43 is known to have excellent and well-understood mechanical properties. In addition, such magnesium alloy is already being widely used in the aerospace industry due to its outstanding mechanical properties [13].
Mechanical Properties | Requirements for Wheel Production | Magnesium Alloys | ||||||||||||||
AM100 | AS41 | AZ31 | AZ61 | AZ80 | AZ81 | AZ91 | EZ33 | HK31 | ZE41 | ZK60 | AM20 | AM60 | AM70 | WE43 | ||
Specific Strength (MPa*cc/g) | Minimum 80 MPa*cc/g | 144.75 | 118.64 | 146.89 | 172.22 | 191.67 | 153.33 | 127.07 | 76.50 | 145.25 | 112.64 | 196.72 | 117.98 | 133.33 | N/A | 135.87 |
Ductility (Elongation) | Minimum 7% | 7% | 6% | 15% | 16% | 5% | 15% | 3% | 2% | 21% | 5% | 12% | 20% | 13% | N/A | 7% |
Fatigue Strength (MPa) | Minimum75 MPa | 70 | N/A | 120 | 125 | 100 | 76 | 95 | 100 | 70 | 130 | N/A | N/A | N/A | N/A | N/A |
Impact Strength (J) | Minimum 3 J | 2.0 | 2.5 | 3.2 | 2.2 | N/A | 4.5 | 1.3 | 0.8 | 3.0 | 1.4 | N/A | 4.2 | 3.0 | 1.8 | N/A |
Corrosion Rate (mg/cm2/day) | Minimum 0.64 (mg/cm2/day) | N/A | 0.25 | N/A | N/A | N/A | N/A | 0.63 | N/A | N/A | N/A | N/A | N/A | 0.13 | N/A | N/A |
Galvanic Corrosion | Zero tolerance | Satisfied | Satisfied | Satisfied | Satisfied | Satisfied | Satisfied | Satisfied | Satisfied | Satisfied | Satisfied | Satisfied | Satisfied | Satisfied | Satisfied | Satisfied |
Tensile Strength (MPa) | N/A | 262 | 210 | 260 | 310 | 345 | 276 | 230 | 140 | 260 | 205 | 360 | 210 | 240 | N/A | 250 |
Yield Strength (MPa) | N/A | 124 | 140 | 200 | 230 | 250 | 85 | 169 | 95 | 195 | 140 | 295 | 90 | 130 | N/A | 162 |
Density (g/cc) | N/A | 1.81 | 1.77 | 1.77 | 1.80 | 1.80 | 1.80 | 1.81 | 1.83 | 1.79 | 1.82 | 1.83 | 1.78 | 1.80 | N/A | 1.84 |
Table 2. List of potential magnesium alloys suitable for wheels production [8] [9].
AZ31 | AZ91 | ZK60 | AM20 | WE43 | |
Costs | CAD$3.24/kg | CAD$3.52/kg | CAD$9.16/kg | CAD$4.30/kg | CAD$3.42/kg |
OtherCharacteristics | Good forgeability, medium strength, can be hammer forged, seldom used | Excellent castability, good strength | Good forgeability,good strength, good ductility | High ductility and impact strength | Fine grained and pressure tight with good casting characteristics |
Typical Applications | Forgings and extruded bar, rod, shapes, structural sections, and tubing with moderate mechanical properties and high elongations | Usually used for die-casting | Extruded products and press forgings, with high strength and ductilityHigh mechanical properties, problem with hot shortness, cracking, not good for sand/permanent mould castings, not weldable, has long fatigue lifeMore costly than AZ series, zirconium is a strong grain refiner, it precipitates iron contaminates from alloy before castingMore than 2.7% to 3% Zn, lose corrosion resistance, hot tearing is not a problem in HPDC | Usually used for die casting | Forgings and extruded bars, excellent retention of properties at elevated temperatures and good corrosion resistance |
Table 3. Costs and other characteristics of the remaining alloy candidates [9] [12].
Magnesium alloy WE43 is a strong casting or forging magnesium based alloy. Its operating temperatures can reach up to 300˚C. The excellent retention of mechanical properties at elevated temperatures, as well as the enhanced corrosion resistance of such alloy attracts designers in the aerospace industry and in the automotive industry [9]. The chemical compositions of the magnesium alloy WE43 are shown in Table 4 below.
In terms of machining, WE43 machines fairly fast compared to any other metal. The power required per cubic centimeter of metal machined varies anywhere from 9 to 14 watts per minute, but this is highly dependent on the operation performed. In terms of surface treatment, WE43, which contains about 4% yttrium, do not respond to certain chemical treatments; for instance, some of the chromate conversion coating baths. In terms of corrosion resistance, due to the presence of yttrium, WE43 exhibits excellent corrosion resistance characteristics [14].
4. Manufacturing Process Selection
Several potential manufacturing processes for magnesium alloy road wheels exist, namely high pressure die-casting (HPDC), low pressure die-casting (LPDC), liquid forging, solid forging, gravity casting, and sand casting. Detailed studies of the latter manufacturing methods are conducted below, and the best one is selected for the production of magnesium WE43 alloy wheels.
4.1. General Die-Casting Process
General die-casting is known to be an efficient and economical process that is able to offer a broader range of shapes than any other manufacturing technique [10]. In addition, die-casting products are known to have a long service life and they require little or no machining. Die-casting is a high speed production process due to the fact that it allows thousands of identical castings to be produced. Tables 5-9 show the typical properties of most commonly used alloys in general die-casting, the characteristics of die-casting alloys, the dimensional, weight limit, and finishing for die-casting alloys, aesthetic surface finishing of die-casting alloys, and the comparison of die-casting with other manufacturing processes, respectively.
Yttrium | 3.7% - 4.3% |
Rare Earths* | 2.4% - 4.4% |
Zirconium | 0.4% minimum |
Magnesium | 90.9% - 93.5% |
Table 4. Chemical compositions of magnesium alloy WE43 [13].
*Rare earths consist of neodymium 2.0% - 2.5%, the remainder being heavy rare earths, principally ytterbium, erbium, dysprosium, and gadolinium.
Aluminum | Brass | Magnesium | Zinc | |
Tensile strength, MPa | 323.83 | 378.95 | 234.26 | 282.49 |
Yield strength, MPa (0.2% offset) | 15.85 | 20.67 | 15.85 | - |
Shear strength, MPa | 192.92 | 254.93 | 137.8 | 213.59 |
Fatigue strength, MPa | 137.8 | 172.25 | 96.46 | 48.23 |
Elongation % (0.05 m samples) | 3.50 | 15 | 3.0 | 10 |
Hardness (Brinell) | 80 | 91 | 63 | 82 |
Specific gravity (SG) | 2.71 | 8.30 | 1.80 | 6.60 |
Weight, kg/m3 | 2712.6 | 8442.4 | 1826.9 | 6643.2 |
Melting point (liquid), ˚C | 1922.4 | 2948.4 | 1931.4 | 1252.8 |
Thermal conductivity, W/m·K | 0.23 | 0.21 | 0.16 | 0.27 |
Impact strength (Charpy), m/kg | 2.02 | 26.89 | 1.34 | 28.91 |
Table 5. Typical properties of most commonly used alloys in general die-casting [10].
Aluminum | Brass | Magnesium | Zinc | |
Dimensional stability | Good | Excellent | Excellent | Good |
Corrosion resistance | Good | Excellent | Fair | Fair |
Casting ease | Good | Fair | Good | Excellent |
Part complexity | Good | Fair | Good | Excellent |
Dimensional accuracy | Good | Fair | Excellent | Excellent |
Die cost | Medium | High | Medium | Low |
Machining cost | Low | Medium | Low | Low |
Finishing cost | Medium | Low | High | Low |
Table 6. Characteristics of die-casting alloys [10].
Aluminum | Brass | Magnesium | Zinc | |
Maximum weight, kg | 31.75 | 4.54 | 19.96 | 34.02 |
Minimum wall thickness, large casting, cm | 0.203 | 0.229 | 0.254 | 0.0889 |
Minimum wall thickness, small casting, cm | 0.102 | 0.140 | 0.102 | 0.0381 |
Cored holes minimum diameter, cm | 0.203 | 0.635 | 0.203 | 0.127 |
Table 7. Dimensional, weight limit, and finishing for die-casting alloys [10].
Aluminum | Brass | Magnesium | Zinc | |
Chrome plating | Fair | Excellent | Fair | Excellent |
Black chrome plating | Fair | Excellent | - | Excellent |
Colored plating | Fair | - | - | Excellent |
Mechanical-polishing & buffing | Excellent | Excellent | Excellent | Excellent |
Lacquers, enamels, epoxies & acrylics | Excellent | Excellent | Excellent | Excellent |
Anodizing | Fair | - | - | - |
Table 8. Aesthetic surface finishing of die-casting alloys [10].
Gravity Casting | Die Casting | Solid Forging | Liquid Forging | |
Economy | C | A | D | B |
Reliability | C | D | A | B |
Design Flexibility | A | C | D | B |
Complexity | C | A | D | B |
Table 9. Comparison of die-casting with other manufacturing processes.
4.2. High Pressure Die-Casting versus Low Pressure Die-Casting
4.2.1. High Pressure Die-Casting (HPDC)
In the automotive industry, various component parts of magnesium alloys are often manufactured using the high pressure die-casting (HPDC) process. Examples of component parts include: alloy AZ81 for the gearbox casing, alloy AS21 for the crankcase, and alloy AZ91 for various automobile applications [15].
There are two ways to perform the HPDC process: one can either use the HPDC hot chamber process or the HPDC cold chamber process. Generally, the HPDC cold chamber process is used to manufacture large components (i.e.: structural parts), whereas the HPDC hot chamber process is used to manufacture small and thin-walled components [12].
The major advantages of the HPDC process (Figure 1) are its high volume, high efficiency, and low production cost. The major disadvantage of this technique is that the manufactured parts usually carry numerous porosities. Such porosities are caused by the aggressive nature of the process, which in turn induces high levels of turbulence at high velocity during metal injection (due to high pressure). Note that it is due to this high level of turbulence combined with entrapped air that results in porosities. In terms of mechanical properties of a metal, porosities are detrimental to its tensile strength and elongation [10]. In addition, due to the porosities issue, it is difficult to further enhance the mechanical properties of the components by heat treatment. As a result, the application of HPDC components in high-safety and airtight systems is limited. The entrapped porosity will also impact upon other key performance attributes necessary for automotive applications, including durability and pressure tightness [9]. It is clear that further increase in magnesium application in the transport industry will require a major advance in processing technologies. These new processes need to be capable of producing components of high integrity and improved performance while being comparable with the HPDC process in terms of production cost and efficiency.
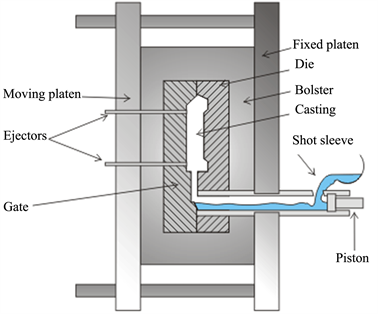
Figure 1. High pressure die-casting [1].
4.2.2. Low Pressure Die-Casting (LPDC)
Low pressure die-casting (Figure 2) was initially developed to improve the quality of gravity die-casting in aluminum and zinc alloys, as well as other metals [8]. The principle of the low pressure die-casting process is as follows. The mould or die is sited above a bath of molten metal in a gas-tight chamber. Then, a riser tube extends from well below the surface of the metal to the base of the mould cavity. Next, metal is constrained to rise smoothly and without turbulence until it completely fills the mould by using the pressure of the atmosphere above the melt (usually less than 69 kN/m) [8]. After that, solidification contraction is provided from the reservoir of molten metal under pressure in the riser tube. Finally, the pressure is released when solidification is fully achieved in the mould, and the molten metal in the tube drains back into the furnace.
4.2.3. Advantages and Disadvantages of High Pressure Die-Casting and Low Pressure Die-Casting
Table 10 below shows the advantages and disadvantages of high pressure die-casting (HPDC) and low pressure die-casting (LPDC).
4.3. Solid Forging
As a general definition, solid forging is a process of working metal to a finished shape by hammering or pressing and is primarily a hot operation. Such technique is mostly employed in the manufacturing of parts that have a rather irregular shape or that are too costly to be done using other methods (i.e.: castings) [16]. Solid forging can be subdivided into many categories, namely open-die forging, closed-die forging, drop forging, press forging, and roll forging. Open-die forging is generally known as hand, smith, hammer, and flat-die forging. The size of a forging that can be produced in open dies is limited only by the capacity of available equipment for heating, handling, and forging. For instance, marine propeller shafts (typically 23 m long) are usually forged by open-die practice, so are forgings no more than a few inches [17]. Open-die forgings may weigh from

Figure 2. Low pressure die-casting [1].
HPDC | LPDC | |
Advantages | 1) High productivity resulting in lower cost2) High volume, high efficiency3) Parts may have complex geometries, exhibiting multifunctional designs4) Cold chamber process suits larger components5) Hot chamber process suits comparatively small and thin-walled components | 1) Prevent molten metal from contacting the air (avoid porosity)2) Lower cost than HPDC(8 - 10 times cheaper)3) Less mould wear than HPDC |
Disadvantages | 1) Substantial amount of porosity: detriment in mechanical properties (i.e.: tensile strength and elongation)2) Less durability and pressure tightness | 1) Labour intensive, need skilled operators |
Applications | 1) AZ81 for gearbox casing2) AS21 for crankcase3) AZ91 for various applications | 1) AZ91 for road car wheels |
Table 10. Advantages and disadvantages of HPDC and LPDC [15].
a kilogram to as much as 300 tons, but usually, open-die forgings (80%) weigh between 14 kg to 454 kg each [17]. Closed-die forging is characterized as the shaping of hot metal completely within the walls or cavities of two dies that come into contact together in order to surround the workpiece on all sides. Closed-die forgings may have complex shapes, and heavy reductions can be made in hot metal within closer dimensional tolerances than are usually feasible with open dies. Drop forging is a technique where the metal is forced with continuous hammering into a metal die. Note that drop forging can be fully automated and is often used for automobile applications. To speed up the production two dies are simultaneously stamped. In press forging, the workable hot metal is slowly compressed into a die. Such technique is mainly used for making tools. Roll forging is a continuous operation in which it compresses hot metal between two die-embedded rollers [15]. Magnesium alloys most commonly solid forged are presented in Table 11 below.
Alloy Type | Alloy Name |
Commercial Alloys | ZK21 |
ZK31 | |
AZ61 | |
High-Strength Alloys | ZK60 |
AZ80 | |
Elevated Temperature Alloys | HM21 |
EK31 | |
Special Alloys (contain nominal 0.6% to 0.7% zirconium) | ZE42 |
ZE62 | |
QE22 |
Table 11. Magnesium alloys commonly solid forged [15].
4.4. Liquid Forging (Squeeze Casting)
In order to compete with and gain a share of the aluminum wheel market, a new and advanced magnesium wheel manufacturing technology should be developed. This technology should fulfill at minimum two requirements. First, it should produce road wheels with productivity and product quality better than that of aluminum wheels. Second, it should produce wheels with considerably increased mechanical properties so that the wall thickness of these wheels can further be reduced resulting in even lighter and less expensive products. Liquid forging, also known as squeeze casting, is a potential candidate to fulfill these requirements. With this technique, it has been studied that the manufactured liquid forged magnesium wheels would exhibit approximately 50% higher fatigue strength, 4 times higher ductility, 5 times higher impact strength, and 10% higher tensile strength than the low pressure permanent mold casting magnesium wheels [18]. Comparing with the HPDC method, the major advantages of the liquid forging process is the elimination of microporosity (shrinkage and gas porosity), and the minimization of component machining. The drawback of this technique is that no heat-treatment can be done on liquid forged components because such treatment would cause blistering and distortion of the liquid forged parts.
In liquid forging, a specific amount of molten metal is required and is injected into a preheated and lubricated bottom die. The molten metal is forged as the upper die closes the bottom half and squeezes the molten metal during solidification. With a combination of gravity die-casting and closed die forging, the metal solidifies under the direct action of pressure [17]. The latter pressure remains present until freezing is complete. Liquid forging is an excellent technique for producing pressure-sealed, low porosity, weldable components with high quality reproducibility. In addition, such technique increases a component part’s strength and ductility with its fine-grained microstructure. Figure 3 below shows the steps of the liquid forging method: 1) proportioning, 2) swinging to the vertical axis, 3) hooking up to the mould, and 4) mould-filling and freezing.
Liquid forging can be further subdivided into two different categories, namely direct liquid forging and indirect liquid forging. In the former technique (Figure 4), the pressure is applied to the entire surface of the liquid metal during freezing, producing castings of full density. As a result, direct liquid forging has a rapid heat transfer, yielding exceptionally fine grain structures. Such technique is mostly used for the production of full integrity castings and metal matrix composite (MMC) components. Note also that direct liquid forging does not require any clamping system [6].
In the indirect liquid forging process, metal is injected into the die cavity by a small amount and the quality of cast product is not as good as the one obtained from the direct liquid forging process. This technique is rarely used in mass production of components [6].
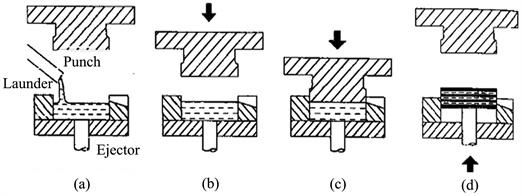
Figure 3. Liquid forging process [6].
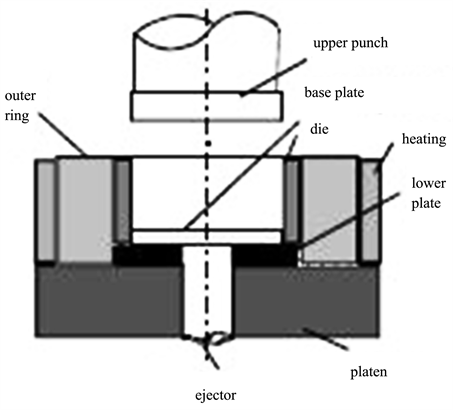
Figure 4. Direct liquid forging [6].
4.5. Gravity Casting and Sand Casting
The way that gravity casting works is simple. It is a basic process that pours molten metal (i.e.: magnesium) into a mold in order to fill it. The manufacturing costs related to gravity casting are fairly low. In addition, such technique allows the castings of various designs (i.e.: complicated geometries). Note that due to the fact that gravity casting makes use of the earth’s gravity to load the mold, the metal is not as densely packed in the mold as some other casting processes [18].
Another casting method is known as sand casting. This technique stabilizes sand that will form all-around a master pattern so as to produce a negative form in which the positive casting results. The tools and equipments cost for sand casting is fairly low, which allows economic production of various castings in different applications. The main drawback of such technique is that it requires more machining than other manufacturing methods due to the fact that the dimensions of the castings are not precise. It is also important to note that sand casting has a relatively slow solidification rate compared to other casting techniques [15].
4.6. Selecting the Manufacturing Process
First, one must select one of the two major manufacturing processes to be used: casting or forging. Since the cost of magnesium wheels forging technology is much higher than that of the casting, only casting manufacturing methods will be considered from here on. Table 12 compares and rates the attributes of each main magnesium wheels casting processes. A score between 0 (lowest) and 5 (highest) is used to rate each attribute. From Table 12, it is clear that low pressure die-casting (LPDC) is the best choice of manufacturing process, with a total and highest score of 41 compared to the other methods.
Attribute | Sand Casting | Gravity die Casting | Low Pressure Die Casting | High Pressure Die Casting | ||||
Potential Casting Soundness | High | 4 | High | 4 | High | 5 | Low | 2 |
Mould Filling | Turbulent | 2 | Turbulent | 2 | Smooth Bottom-filled | 5 | Very turbulent | 0 |
Cover Gas Management | Difficult | 2 | Difficult | 2 | Difficult and costly | 1 | Easy | 5 |
Sand Cores for Hollow Features | Yes | 5 | Yes | 5 | Yes | 5 | No | 0 |
Typical Casting Yield (%) | 50% | 1 | 60% | 2 | 85% | 4 | 60% | 2 |
Cycle time | Very Long | 0 | Long | 2 | Medium | 3 | Short | 5 |
Heat Treatable Castings | Yes | 5 | Yes | 5 | Yes | 5 | No | 1 |
Weldable Castings | Yes | 5 | Yes | 5 | Yes | 5 | No | 0 |
Operating Cost | Low | 5 | Low | 5 | Medium | 2 | Medium | 2 |
Tooling Cost | Low | 5 | Low | 3 | Low/Med | 3 | High | 1 |
Machine Cost | Very Low | 5 | Low | 4 | Low/Med | 3 | Very High | 0 |
Total Score | 39 | 39 | 41 | 18 |
Table 12. Casting manufacturing processes comparison table [15] [18].
The low pressure die-casting machine (supplier: Liujin Foundry Machinery Works Co. Ltd.) has decided to be purchased due to low purchasing costs and low energy consumption. The machine model is J452-B (Figure 5). The cost of the machine is CAD$34,000, and it can be used to cast either aluminum or magnesium road wheels [19]. The total power consumption of the machine is 28 kWh.
5. Important Properties to Consider on the Low Pressure Die-Cast Magnesium Alloy WE43 Wheel
Before and after the low pressure die-cast magnesium alloy WE43 wheel is manufactured, there are several important properties to consider before such wheel can be safely used on the road. These properties include the pressure tightness, the corrosion resistance, and the fatigue strength.
5.1. Pressure Tightness
The definition of pressure tightness is the resistance to leakage through casting under pressure. The meaning more or less implies that if a metal component is pressurized the cast should maintain the force exerted on the material walls without seeping out [21].
The result of a cast is greatly related on how the product is designed, the layout of the tooling (mould) and the process control during the actual casting. All these aspects play a large role in the pressure tightness of the casting. With excellent properties as mentioned it should be possible to make pressure tight casting from any alloy [3].
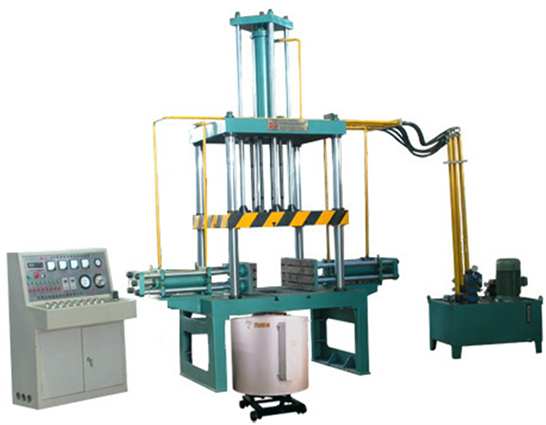
Figure 5. Low pressure die-casting machine (model J452-B) [20].
To test if a casting is pressure tight and leakage is not present the simplest method is to close all openings and pressurize the interior. Immersing this pressurized system into water will reveal any sources of pressure leakage. If no bubbles are generated the casting is pressure tight. It is apparent that the key element to a leak in a system is porosity. On the other hand a pressure tight casting does not signify a pore free product but very close to one. Porosity may still exist but not in a manner where the gas/liquid can channel through from one side to the other.
In general, two categories of porosities exist in metal castings: macro-porosity and micro-porosity. Macro-porosities are defined by large flaws that are visible to the naked eye and micro-porosities are voids barely visible. These porosities irregardless of macro or micro may exist in two distinct manners: continuous porosity and blind porosity [4]. Figure 6 demonstrates the two in liquid and powder casting.
Blind or enclosed porosity are voids that are not interconnected within the part while continuous porosity may stretch from one end of the part to the other allowing the pressurized gas or liquid to escape.
Pressure tightness testing whether hydraulic for large pieces or gas pressure for small parts does, however, give an indication of the quality of the cast, revealing defects which might have significant or even insignificant effects on the strength of the casting. The manner in which pressure tightness plays a role in magnesium wheels is such. If a product is pressure tight it does give the indication of higher mechanical properties than that which is not pressure tight. Therefore with higher mechanical properties allows greater chance of reduced section thickness and thus further weight reductions. Furthermore, a part that is lacking pressure tightness may lose plating and finishing quality, since the surface pores may cause bleedout or spotting of the plating, coating, and/or finishing solutions. Evidently this phenomenon will vary the life of the wheel [9].
A solution to the deficient pressure tightness in a casting is through impregnation. Impregnation refers to the sealing of the leaks caused by the porosity. Impregnating material, usually a liquid is introduced into the voids on both sides of the casting wall via vacuum and pressure. Figure 7 shows the difference between a part with and without the use of impregnating material prior to coating.
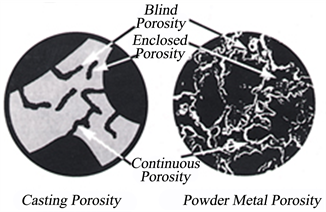
Figure 6. Continuous and blind porosity [4].
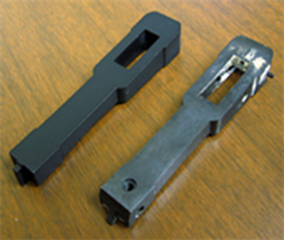
Figure 7. Part on left shows impregnated coated part and part on right shows breakdown of coating without impregnation [4].
Castings with continuous or blind porosity can have improved surface finish by being impregnated prior to coating application. Another advantage of impregnating the wheel is for pressure tight parts that contain temporary blind porosity. A part can be tested for pressure tightness and pass. But after slight machining or going through a thermal shock during its lifetime that blind porosity may become a continuous porosity [15]. The only difference between the two porosities is a membrane that prevents the channels from being complete. Subsequently, machining or a thermal shock will easily destroy this membrane taking away all benefits of a pressure tight system.
It is evident that pressure tightness plays a one-time role in the manufacturing process of the magnesium alloy wheel WE43. At the beginning of the process the pressure tightness of the cast should be tested and corrected accordingly with an appropriate safety factor. Once this is done it will not be necessary to recheck this trait until the mould or a main casting parameter is changed.
5.2. Corrosion Resistance
Magnesium alloy WE43 has a fairly high corrosion resistance. This is mainly due to the yttrium addition as alloying element (approximately 4%). Figure 8 below shows the corrosion rate in mg/cm2/day versus the different constituents in weight %. It can be seen that constituents of aluminum, silicon, manganese, yttrium, and rare earths all contribute to a high corrosion resistance.
In order to further enhance the corrosion resistance, one may apply a protective coating on the metal alloy. There are two different types of coatings that are used in road wheels: the anodic coatings and the chemical conversion coatings. The anodic coatings are tougher, harder, and have better wear properties compared to chemical conversion coatings. The chemical conversion coatings are based on the generation of sparks on the alloy surface to develop ceramic-like films. Due to today’s strict environmental regulations, the use of chromate-based coatings is limited. Currently, research is being done on the development of chromate-free coating methods. Typical coating thickness of such method is approximately tens of micrometers [6].

Figure 8. Corrosion rate versus constituent [1].
5.3. Fatigue Strength
Fatigue is defined as the progressive and localized structural damage which occurs when a given material is subjected to cyclic loadings. Usually a car is designed for a life of 300,000 km. The highest numbers of load cycles usually occur at the wheel and at those parts that are subjected to engine vibrations. Road wheels experience two load cycles per revolution. This results in 3 × 108 load cycles for a 15" (38.1 cm) diameter wheel after 300,000 km [22]. The excitation from the engine vibrations may cause a number of load cycles well above 109, depending on the number of engine-order and gearbox transmission. Those high numbers of load cycles cannot be reached in reasonable time with resonant or servo-hydraulic machines [9]. Because the wheels that were designed based on this criterion have been used safely for a long time, it can be said that they have enough fatigue strength for conventional loading conditions. Recently, however, a demand has risen for reducing the weight of the wheel to increase the speed of the vehicle, and also for saving the fuels and reducing the pollutant gases emissions [18]. When designing a new wheel such as magnesium wheel for use under severer conditions, it is necessary to review the fatigue safety of the existing wheels (e.g. steel or aluminum wheels), and to achieve the equivalent fatigue strength in the new wheel. Therefore the investigations on fatigue life estimation for the wheels are necessary and useful.
For a more general description of magnesium alloy WE43, please refer to Section 3. Table 13 below shows the ambient temperature mechanical properties of LPDC magnesium alloy WE43.
As one can see from Figure 9 below, WE43 shows excellent fatigue properties. The maximum stress that WE43 can stand after cycles of stress of up to 108 remains at approximately 80 MPa.
Figures 10-13 below show the elevated temperature mechanical properties of LPDC magnesium alloy WE43.
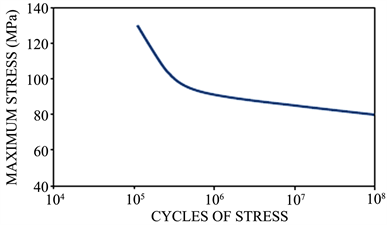
Figure 9. Rotating bend fatigue test [13].
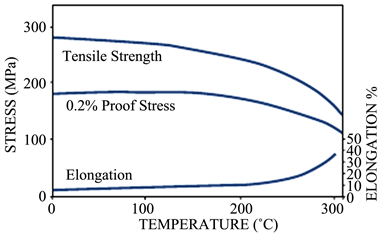
Figure 10. Effect of temperature on tensile properties [13].
Typical Tensile Properties | |
0.2% Proof Stress | 180 MPa |
Tensile Strength | 250 MPa |
Elongation | 7% |
Typical Compressive Properties | |
0.2% Proof Stress | 187 MPa |
Ultimate Stress | 323 MPa |
Typical Shear Properties | |
Ultimate Stress | 162 MPa |
Fracture Toughness | |
KIC | 15.9 MPa m1/2 |
Fatigue Properties (see Figure 14 below) |
Table 13. Ambient temperature mechanical properties of LPDC Mg alloy WE43 [13].
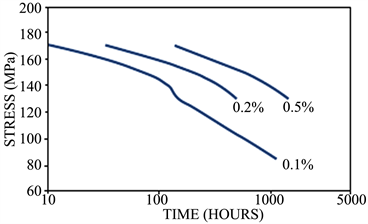
Figure 11. Stress/time relationship for specified creep strains at 2000˚C [13].
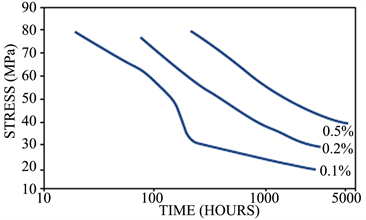
Figure 12. Stress/time relationship for specified creep strains at 2500˚C [13].
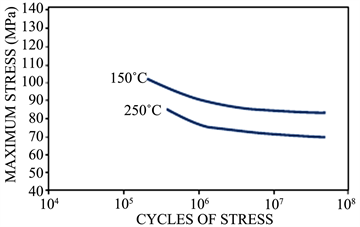
Figure 13. Rotating bend fatigue test [13].
It can be seen that the tensile strength of WE43 remains relatively constant at 280 MPa even at a temperature of up to 2000˚C, after which the strength decreases to about 180 MPa.
Even with increasing temperature, it can be noted that the fatigue strength of WE43 does not decrease dramatically (only slight decrease in fatigue strength with increasing temperatures). This is important in road wheels due to the high temperature effects on the wheels during breaking.
The microstructure of a LPDC magnesium alloy WE43 is shown in Figure 14 below. It can be seen that the major alloying elements are concentrated at grain boundaries [23].
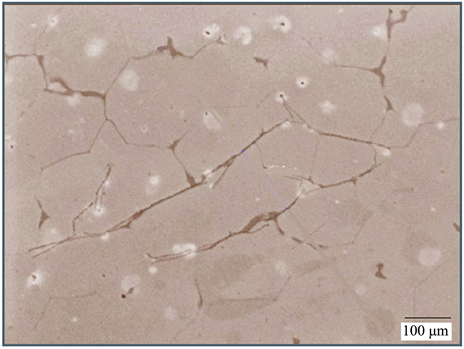
Figure 14. Microstructure of magnesium alloy WE43 [23].
7. Cost Analysis/Economic Viability
7.1. Cost Analysis
The economic viability and cost analysis were performed by comparing the estimated total cost per the manufacturing of one wheel between a commonly used aluminum alloy wheel (A356, LPDC) and the magnesium alloy wheel (WE43, LPDC). The first step is to determine the metal cost per wheel based on the base metal cost and weight of the wheel (17 inch (43 cm) diameter). Note that the metal used for the manufacturing of one wheel takes into account a 20% of scrap material given the fact that some of the material may be lost during the processing stages. Then, based on the power consumption of the low pressure die-casting machine (note that the same machine can be used both for the manufacturing of aluminum wheels and magnesium wheels) and the power price charges (set by Hydro-Quebec) [24], the cost of power per wheel is determined, assuming a production of 15 wheels per hour. It is important to remember that the number of wheels produced per hour would not affect the final total manufacturing cost per wheel because the same machine (hence, same power consumption) is being used for both alloys. Next, assuming a total of 4 casting operators with an annual salary of CAD$35,000 each, the labor cost per wheel is determined. In addition, it has been assumed that the manufacturing plant operates 8 hours a day, 5 days a week, and 52 weeks a year. Finally, the total estimated cost per wheel for both types of alloy is obtained by adding all previously described costs. The results of the cost analysis for the manufacturing of one wheel are shown in Table 14 below. IMPORTANT NOTE: the cost analysis and economic viability studies performed in this section take into account all costs related to the manufacturing of the wheel only. The analyses do not include building costs (manufacturing plant, land, site development, services, offices, and laboratories), nor do they include labor costs other than casting operators
Aluminum Alloy A356 Wheel (LPDC) | Magnesium Alloy WE43 Wheel (LPDC) | Magnesium Alloy WE43 Wheel (LPDC) | |
Base Metal Costs | CAD$2.20/kg | CAD$3.42/kg (China) | CAD$80/kg (UK) |
Metal Used/Wheel (including an assumption of 20% of scrap material) | 10.92 kg (17" (43 cm) diameter) | 8.19 kg (17" (43 cm) diameter) | 8.19 kg (17" (43 cm) diameter) |
Metal Costs/Wheel | CAD$24.02 | CAD$28.01 | CAD$655.20 |
Power Consumption of LPDC Machine | 28 kWh | 28 kWh | 28 kWh |
Power Price Charges | Fixed Charge CAD$12.33 (30 days)Energy Charge CAD$8.47¢/kWh | Fixed Charge CAD$12.33 (30 days)Energy Charge CAD$8.47¢/kWh | Fixed Charge CAD$12.33 (30 days)Energy Charge CAD$8.47¢/kWh |
Cost of Power/Hour (assuming 8 hours operation per day) | CAD$7.17 | CAD$7.17 | CAD$7.17 |
Cost of Power/Wheel (assuming a production of 15 wheels an hour) | CAD$0.48 | CAD$0.48 | CAD$0.48 |
Labor Cost/Hour (assuming 4 casting operators with an annual salary of CAD$35,000 each) | CAD$67.31 | CAD$67.31 | CAD$67.31 |
Labor Cost/Wheel (assuming a production of 15 wheels an hour) | CAD$4.49 | CAD$4.49 | CAD$4.49 |
Estimated Cost/Wheel | CAD$28.99 | CAD$32.98 | CAD$660.17 |
Table 14. Cost analysis for the manufacturing of one wheel.
(president, secretary, sales manager, security, production engineer, coordinate measuring operator, quality control inspectors, etc.). In addition, for the sake of base metal price comparison, the following cost analysis includes the manufacturing of one wheel using a magnesium alloy WE43 imported from China, and another wheel using a magnesium alloy WE43 imported from UK. It can be seen that the manufacturing cost of one magnesium wheel using WE43 imported from UK is too expensive compared to the one using WE43 imported from China, hence, the subsequent analyses in this Section will ignore the magnesium alloy imported from UK.
It can be seen that the estimated manufacturing cost per one wheel is higher for magnesium than that of aluminum. However, this cost difference is not dramatic (CAD$3.99, ~12% difference) and can be justified by the enhanced mechanical properties of magnesium alloy WE43 rather than those of a commonly used aluminum alloy (refer to Sections 3 and 6 for detailed analysis on the mechanical properties of magnesium alloy WE43).
7.2. Economic Viability
According to the theories of engineering economics, the Benefit to Cost Ratio (BCR) must be higher than unity in order for a given project to be profitable [25]. A BCR is an indicator, used in the formal discipline of cost-benefit analysis, which attempts to summarize the overall value for money of a project or proposal [26]. The latter indicator is the ratio of the benefits of a project or proposal, expressed in monetary terms, relative to its costs, also expressed in monetary terms. All benefits and costs are expressed in discounted present values. From Table 15 and based on the theory of the BCR, it can be seen that the selling price of one magnesium alloy WE43 wheel must be higher or equal to CAD$40.
7.3. Proposed Financing
The proposed financing is mainly based on partner search and marketing (including the search of customers for magnesium wheels). The potential partners/customers are presented in the next Section.
7.4. Potential Customers
At first glance, Japanese car companies seem promising in terms of supplying a customer base for the magnesium alloy WE43 wheels due to their large market around the world. Unfortunately, Japanese car companies have proven to be fairly conservative in terms of investing in new wheel technologies [27]. This is not the case for American companies. For instance, Daimler Chrysler has recently showed interest in developing magnesium wheels. In fact, the Chevrolet Corvette models already use magnesium wheels so as to decrease the car weight and to enhance the steering capabilities of the vehicle [27]. Hence, Daimler Chrysler could be a potential customer that would be interested in subcontracting magnesium alloy WE43 wheels. Other American companies may also be good potential partners or customers.
7.5. Potential Competitors
The current wheel market is greatly competitive, especially for magnesium alloy wheels that have to compete with steel or aluminum alloy wheels. Fortunately, this project is targeting OEM (original equipment manufacturer) and not AM (after market), the competition is not as high. Nevertheless, most North American wheel manufacturers moved their facilities to developing countries due to cheaper labor cost. In order to be competitive, it must stand out in wheel competitive factors including price, performance, availability, and design/finish.
Scenario 1 | Scenario 2 | Scenario 3 | Scenario 4 | |
Cost/Wheel | CAD$32.98 | CAD$32.98 | CAD$32.98 | CAD$32.98 |
Selling Price/Wheel | CAD$150 | CAD$60 | CAD$40 | CAD$38 |
Profit/Year | CAD$3,651,024 | CAD$843,024 | CAD$219,024 | CAD$156,624 |
Production Cost/Year* | CAD$188913.6 | CAD$188913.6 | CAD$188913.6 | CAD$188913.6 |
BCR | 19.33 | 4.46 | 1.16 | 0.83 |
Table 15. Economic viability analysis.
*The production cost per year includes equipment cost, labor cost, and production cost.
This research project has come up with a proposal to produce magnesium alloy WE43 road wheels by the low pressure die-casting process. Such wheels will have excellent mechanical properties and have shown to be economically viable. Although the current wheel market is dominated by aluminum alloy wheels, we foresee that magnesium alloy wheels will slowly gain their place in the wheel market due to their outstanding properties.
This work will be based on OEM (original equipment manufacturer) wheels designated for all types of vehicles, including sports, prestige, luxury, and every day cars. By reason of the large profitability of the production of magnesium alloy wheels, it may provide opportunities in the future to look into AM (after market) wheels.
Conflicts of Interest
The authors declare no conflicts of interest regarding the publication of this paper.
[1] | Garmo, E.P.D., Black, J.T. and Kohser, R.A. (1997) Magnesium and Magnesium Alloys. In: Materials and Processes in Manufacturing, 8th Edition, Wiley, New York, 112-184. |
[2] | Zhang, Z.L., Zhang, Y.Q. and Zhang, L. (2015) Application of Micro-Arc Oxidation Technology in Die Magnesium Alloy Wheels in Mass Production. Key Engineering Materials, 667, 15-21. https://doi.org/10.4028/www.scientific.net/KEM.667.15 |
[3] | Avedesian, M.M. and Baker, H. (1999) ASM Specialty Handbook, Magnesium and Magnesium Alloys. |
[4] | Polmear, I.J. (1996) Light Alloys: Metallurgy of the Light Metals. 3rd Edition, Halsted Press, New York. |
[5] | Deborah, A.K. (2001) Magnesium, Its Alloys and Compounds. U.S. Geological Survey Open-File Report 01-341. |
[6] | Smith, W.F. (1993) Structure and Properties of Engineering Alloys. 2rd Edition, McGraw-Hill, New York. |
[7] | Li, G. (2014) Study on Superplastic Forming of Preformed Blank for Magnesium Alloy Wheel. Forging & Stamping Technology, 39, 71-74. |
[8] | Aghion, E. and Bronfin, B. (2000) Magnesium Alloys Development towards the 21st Century. Materials Science Forum, 350, 19-30. https://doi.org/10.4028/www.scientific.net/MSF.350-351.19 |
[9] | Kulekci, M.K. (2008) Magnesium and Its Alloys Applications in Automotive Industry. The International Journal of Advanced Manufacturing Technology, 39, 851-865. https://doi.org/10.1007/s00170-007-1279-2 |
[10] | Kainer, K.U. (2004) Rapid Solidification and Special Processes for Processing Magnesium Alloys. In: Kainer, K.U., Ed., Magnesium—Alloys and Technology, Wiley, Hoboken, 164-183. |
[11] | Price on Magnesium and Aluminium (2022). http://www.lightmetals.org/e/documents/LMTinretospect.pdf |
[12] | Kainer, K.U., Dieringa, H., Dietzel, W., Hort, N. and Blawert, C. (2014) The Use of Magnesium Alloys: Past, Present and Future. Proceedings of Magnesium Technology in the Global Age 2014, Montreal, 23-27 September 2014, 127-135. |
[13] | Ignata, S. (2004) Magnesium Alloys (WE43 and ZE41) Characterization for Laser Applications. Applied Surface Science, 233, 382-391. https://doi.org/10.1016/j.apsusc.2004.04.002 |
[14] | Elektron WE43 Datasheet: 467, Magnesium Elektron: Service & Innovation in Magnesium, 2005-2006. |
[15] | Mathaudhu, S.N., Luo, A.A., Neelameggham, N.R., Nyberg, E.A. and Sillekens, W.H. (2014) Advances in Manufacturing Processes for Magnesium Alloys. In: Essential Readings in Magnesium Technology, John Wiley & Sons, Inc., New York, 19-24. https://doi.org/10.1002/9781118859803 |
[16] | Yang, Z., Li, J.P., Zhang, J.X., Lorimer, G.W. and Robson, J. (2008) Review on Research and Development of Magnesium Alloys. Acta Metallurgica Sinica, 21, 313-317. https://doi.org/10.1016/S1006-7191(08)60054-X |
[17] | Graf, M., Ullmann, M. and Kawalla, R. (2014) Influence of Initial State on Forge Ability and Microstructure Development of Magnesium Alloys. Procedia Engineering, 81, 546-551. https://doi.org/10.1016/j.proeng.2014.10.037 |
[18] | Siobhan, F. (2012) An Overview of Magnesium Based Alloys for Aerospace and Automotive Applications. MSc, Rensselaer Polytechnic Institute, Hartford. |
[19] | Gao, Y.R. (2016) Private Communication. Liujin Foundry Machinery Works Co. Ltd., Beijing. |
[20] | http://hkjum262124.51sole.com/companyproduct.htm |
[21] | Kubota, K., Mabuchi, M. and Higashi, K. (1999) Review Processing and Mechanical Properties of Fine-Grained Magnesium Alloys. Journal of Materials Science, 34, 2255-2262. https://doi.org/10.1023/A:1004561205627 |
[22] | Aghion, E., Bronfin, B., Friedrich, H. and Rubinovich, Z. (2004) The Environmental Impact of New Magnesium Alloys on the Transportation Industry. Proceedings of Magnesium Technology, Warrendale, 25 February-1 March 2004, 31-38. |
[23] | Mathaudhu, S.N., Sillekens, W.H., Neelameggham, N.R. and Hort, N. (2012) Microstructure Modeling of Magnesium Alloys for Engineering Property Prediction. In: Magnesium Technology 2012, John Wiley & Sons, Inc., New York, 333-337. https://doi.org/10.1002/9781118359228.ch61 |
[24] | http://www.hydroquebec.com/majorcustomers |
[25] | Bugliosi, M. (2016) Private Communication, Sales Manager, Magnesium Elektron, UK, 21st January. |
[26] | Benefit-Cost Ratio. http://en.wikipedia.org/wiki/Benefit-Cost_Ratio |
[27] | Ehrenberger, S., Schmid, S. and Friedrich, H. (2008) Magnesium Production and Automotive Applications: Life-Cycle Analysis Focusing on Greenhouse Gases, 16. Magnesium Abnehmer-und Automotive Seminar 2008, Aalen, 11-17 September 2008, 31-35. |