ABSTRACT
This thesis describes, in a manufacturing context, the development of new waterbased core technology for light alloys. Cores used for steel casting are made from fused silica and are removed using hot sodium hydroxide under refluxing (pressurising hot acids). However, aluminium and other light alloys are attacked by sodium hydroxide. Currently there is no good core system for aluminium and other light alloys.
It is therefore desirable to find an alternative material/leaching agent combination for casting aluminium and other light alloys. The recent research review has shown that ceramic cores are mostly made by fused silica with different additives. The previous research has suggested using fused silica (different mesh size) as filling material and using magnesium oxide to control the slurry working life of core mixes.
Calcium silicate assists core leaching in dilute acid. The plaster (calcium sulphate) in the form of proprietary plasters (Crystcal R, Fine Casting Plaster) is used to create bond and gives strength to the core. Lithium carbonate acts has an accelerator, improving the strengthening effect of the plaster in the cores. The binder (Ludox® AM) and water act (as added materials) to bind the composition Core compositions were made with different core trials to produce a core, which records suitable strength and quick leaching properties for light alloys.
Core trials were individually mixed and poured into a wooden core box. Cores were pre-dried for by two hours cooling. The cores were subjected to computerised three-point bend test to record the Modulus of rupture (MOR). The plain strain fracture toughness and Weibull parameters were calculated. The Weibull parameter was plotted using Minitab analysis software. Using the cores, gravity die casting process were carried out.
The subsequent castings was dipped in diluted nitric, citric and acetic acid to leach out the core. Using different core compositions, core trials were mixed, poured, dried, tested and leached. The high amount of plaster in core trials records high MOR when cores were fired between 200°- 400° C and the opposite result when fired between 600°- 800° C. The different grade of plasters(CRP,FCP) do not influence the strength. One percent of magnesium oxide gives a very short working life.
High amount of binder(Ludox® Workable MOR results can be obtained depending on composition allowing manual handling or a waxing process. The fracture toughness is typical of a brittle material, with matching Weibull parameters. The casting process suggests that the new materials are sufficiently refractory.
The cores are leached out using diluted nitric, acetic and citric acid at rates compatible with commercial manufacture. This methodology has successfully produced a core using fused silica with plaster and magnesium oxide for aluminium and possibly for light alloys. Different core trials can be used depending on the specific industrial application relating to strength and removal with acid attacking the metal.
Further work is needed to fine tune optimum leaching conditions. AM) in core produce strong cores. twenty-four hours. Cores were fired to different temperatures for two hours, followed
Korea Abstract
이 논문은 제조 맥락에서 경합금을 위한 새로운 수성 핵심 기술의 개발을 설명합니다. 강철 주조에 사용되는 코어는 용융 실리카로 만들어지며 환류(고온 산 가압) 하에 뜨거운 수산화나트륨을 사용하여 제거됩니다.
그러나 알루미늄 및 기타 경합금은 수산화나트륨의 공격을 받습니다. 현재 알루미늄 및 기타 경합금을 위한 좋은 코어 시스템은 없습니다. 따라서 알루미늄 및 기타 경합금 주조를 위한 대체 재료/침출제 조합을 찾는 것이 바람직합니다. 최근 연구 검토에 따르면 세라믹 코어는 대부분 다른 첨가제를 사용하여 용융 실리카로 만들어집니다.
이전 연구에서는 용융 실리카(다른 메쉬 크기)를 충전재로 사용하고 산화마그네슘을 사용하여 코어 믹스의 슬러리 작업 수명을 제어할 것을 제안했습니다. 규산칼슘은 묽은 산에서 코어 침출을 돕습니다. 전용 플라스터(Crystcal R, Fine Casting Plaster) 형태의 플라스터(황산칼슘)는 결합을 생성하고 코어에 강도를 부여하는 데 사용됩니다.
탄산리튬은 촉진제를 가지고 있어 코어의 석고 강화 효과를 향상시킵니다. 결합제(Ludox® AM)와 물은 조성을 결합하기 위해 작용합니다(추가된 재료로서) 코어 조성은 경합금에 적합한 강도와 빠른 침출 특성을 기록하는 코어를 생산하기 위해 다양한 코어 시도로 만들어졌습니다.
코어 시험을 개별적으로 혼합하고 나무 코어 상자에 부었습니다. 코어는 2시간 냉각 동안 사전 건조되었습니다. 코어는 파열 계수(MOR)를 기록하기 위해 컴퓨터화된 3점 굽힘 테스트를 거쳤습니다. 일반 변형 파괴 인성 및 Weibull 매개변수가 계산되었습니다. Minitab 분석 소프트웨어를 사용하여 Weibull 매개변수를 플로팅했습니다.
코어를 이용하여 중력 다이캐스팅 공정을 진행하였다. 후속 주조물을 희석된 질산, 시트르산 및 아세트산에 담그어 코어를 침출시켰다. 다양한 코어 조성을 사용하여 코어 시험을 혼합, 붓고, 건조하고, 시험하고, 침출했습니다. 코어 시험에서 많은 양의 석고는 코어가 200°-400°C 사이에서 소성될 때 높은 MOR을 기록하고 600°-800°C 사이에서 소성될 때 반대 결과를 기록합니다.
다른 등급의 석고(CRP,FCP)는 영향을 미치지 않습니다. 힘. 산화마그네슘의 1%는 작업 수명이 매우 짧습니다. 많은 양의 결합제(Ludox® Workable MOR 결과는 수동 취급 또는 왁스 처리가 가능한 조성에 따라 얻을 수 있습니다. 파괴 인성은 Weibull 매개변수와 일치하는 취성 재료의 전형입니다.
주조 공정은 새로운 재료가 충분히 내화성이 있음을 시사합니다. 코어는 상용 제조와 호환되는 속도로 희석된 질산, 아세트산 및 시트르산을 사용하여 침출됩니다. 이 방법은 알루미늄 및 가능한 경합금용 석고 및 산화마그네슘과 용융 실리카를 사용하여 코어를 성공적으로 생성했습니다.
다양한 코어 시험을 사용할 수 있습니다. 강도 및 금속을 공격하는 산 제거와 관련된 특정 산업 적용에 따라 최적의 침출 조건을 미세 조정하려면 추가 작업이 필요합니다. 코어의 AM)은 강한 코어를 생성합니다. 24시간. 코어는 2시간 동안 다른 온도로 소성되었습니다.
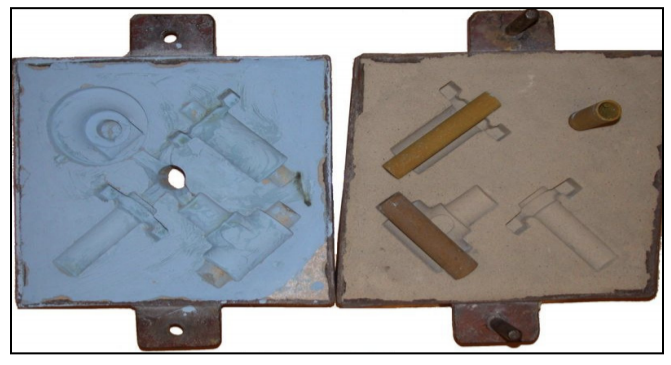
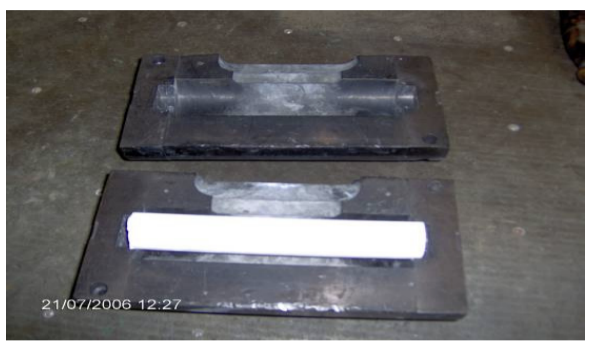
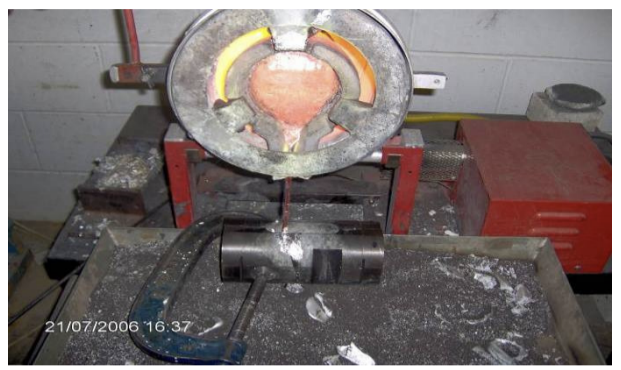
REFERENCES
- CAD Casting: Direct Fabrication of Ceramic Shells and Cores by ThreeDimensional Printing, Sachs E B, Curodeau A D, Manufacturing Review (USA). Volume 5, p117-126, June 1992.
- Ceramic slurry composition for ceramic core of a dental prosthesis, Kim J D, Lee M H, Korean Science Technology, US patent 5975905, November 1999.
- Moulded ceramic cores for the production of precision cast impellers by the unicast process, Greenwood R E, Precision casting supplements, Foundry trade journal, p178-182, March 1984.
- Elements of material science and engineering, Van Vlack L H, Third edition, Addison- Wesley Publishing Company, 1975.
- Ceramic cores in the sand foundry, Greenwood R E, Cast metal times, p11-12, April 2002.
- Casting solutions Ltd, Robson B, Ceramic core and mould expert, Sheffield, February 2004.
- Principles of foundry technology, Jain P L, Second edtion, Tata McGrawHill, 1996.
- Ferrous foundry-man’s handbook, Brown J, Elsevier, p18-20, July 2000.
- www.eos-gmbh.de/030356_appl_cm06.htm, 18 September 2006.
- http://www.core-tech-inc.com/form_ground.html, 18 September 2006.
- Properties of ceramic cores, Mills D, The British investment casters technical association, Fifteenth annual conference, 1980.
- Principle of metal casting, Heine R W, Loper C R, Philip C, Second edition, Tata McGraw-Hill, New Delhi,p121-175, 1976.
- http://www.enercombustion.com/EnServices.htm,19 September 2006.
- Methods of firing core, Altoonian M A, Runions R D, US patent 6403020-B1, p1-7, June 2002.
- Ceramic core; Nucleus for precision internal section, Greenwood R E, Unicast development corporation, Modern technology, p29-31, 1985.
- Alumina-based ceramics for core materials, Greskovich C D, US patent 4156614, GE company, p1-4, October 1977.
- Low temperature co-fired ceramic with improved registration, Shepherd P N, US patent 6560860-B2, CTS Corporation, p 1-6, January 2001.
- Injection molding of ceramic components provides flexibility in metal casting and forming, Uram S, Industrial heating, p42-43, 1987.
- Multipiece core assembly for cast airfoil, Sikkenga W E, Grumm A W, Howmet research corporation, US patent 6347660, February 2002.
- The effect of cristobalite seed on the crystallization of fused silica based ceramic core- A kinetic study, Wang L Y, ceramic international, p187-193, 1995.
- Printed materials on manufacturing fused silica, Minco Company, Midway, TN, USA, 2004.
- Dimensional changes and creep of silica core ceramics used in investment casting of supper alloys, Wereszczak A A, Breder K, Journal of material science, p 4235-4245, April 2002.
- Alumina core having a high degree of porosity and crushability characteristics, Klung F J, US patent 4164424, GE company, p1-12, August 1979.
- Basic inorganic chemistry, Cotton A, Gaus P L, Third Edition, Wiley J &sons Inc, p307-208, 1995.
- Printed material for calcium silicate and Lithium carbonate, Material safety data sheet, Sigma Aldrich Company, Pool,Doorset, UK, 2002.
- A textbook of quantitative inorganic analysis, Vogel A I, Second edition, Longmans, s1939.
- BPB gypsum special plasters, Data sheets on plasters, BS EN 9002:1994. Printed material data sheet for tri-sodium citrate, University of Teesside Chemistry online database, 2005.
- CRC handbook of chemistry and physics, Forty fourth edition, p2292. Ludox AM® , Colloidal silica data sheet, Grace division , Grace W R & Co, Worms, Germany, 2006.
- Water Soluble cores and methods for manufacturing cast rotor, Aiga T, Toshiaki M, US patent 4438804, March1984.
- Basic inorganic chemistry, Cotton A, Gaus P L, Third edition, Wiley J &sons Inc, p231-232, 1995.
- Inorganic Chemistry , An Intermediate Text, Wood C W, Holliday A K, Third Edition, Butterworth’s, 1967,p165-166.
- Standard test method for flexural strength of advanced ceramics at ambient temperature, ASTM Standard C1161.
- Materials handbook, Brady G S, Clauser H R, Vaccari J A, fifteenth edition, McGraw-Hill, 2002.
- http://www.qualitydigest.com/jan99/html/body_weibull.html,19 September 2007.
- Interpret the shape, scale, and threshold on a Weibull probability plot, ID766, Minitab help handbook
- Weibull Analysis, Weibull W, Journal of applied mechanics, p293-297, 1951.
- Engineering materials 2, Ashby M F, Jones D R H, Third edition, Elsevier Publications, p196-197, 2006.
- Strength, fracture toughness and microstructure of a selection of all ceramic materials, Ringer S P, Swain M V, Dental materials, p441-448, June2004.
- Fracture toughness comparison of three test methods with four dental porcelains, Wang H, Pallav P, Dental materials, p905-910, July2007.
- Engineering Materials 2 , Ashby M F, Jones D R H, Third edition, Elsevier Publications, p198-199, 2006.
- Aluminum alloys- structures and properties, Mondolfo L f, Butterworths London and Boston, p971, 1976.
- Aluminum LM25 data sheet, MRT casting limited, Foundries and machinist for non-ferrous cast parts, www.mrt-castings.co.uk., 20th September 2007.
- Pressure die casting part 2, Allsop D F, Kennedy D, Pergamon press, p4-5. 1983.
- Principle of metal casting , Heine R W, Lopper C R, Second Edition, Tata McGraw-Hill Edition, p334-336,1967.
- Casting design handbook, American society for metals, Metals park, p222-223, 1963.
- Gravity die casting process handbook, Shamasundar S, Gopalkrishna V, Ennore foundries limited, Chennai, India, 2002.
- Technology of aluminum and its alloys, Varley P C, Newness International Monographs, p65-66, 1970.
- Pressure die casting-Part 1, Upton B, Buhler M, Pergamon Press,p4-5, 1982.
- Fundamentals in the production and design of castings, Clarence T M, John Wiley & Sons, p1-3, 1950.
- Technology of aluminum and its alloys, Varley P C, Newness International Monographs, p67-68,1970.
- In solidification and casting of metals conference, Herra A, Kondic V, Metal society London, p 460-465, 1979.
- Casting practice, The 10 rules of casting, Campbell J, Elsevier ButterworthHeinemann, p 4 & 114-115, 2004.
- A review of specialist and metal removal techniques, Shaw F M, Garman F K, Fettling and cleaning of castings, University of Nottingham, September 22-24th ,1974.
- Ultrasonic removal of ceramic bars from castings, Sorokin L F, Efimov V M, Energmashinostroenie, p 23, 1988.
- Removal of ceramic cores from investment castings using shock waves, Carden A E, Evces C R, Engel T G, 42nd annual technical meeting, Investment casting institute, , p4:1- 4:4,1994.
- Shock wave process for the removal of mold residues and core for the production of castings, Thewe R, CP & T-Casting plant and technology, Giesserei-Verlag, GmbH, Düsseldorf, Volume 1, p 2, 1993.
- Magnesium oxide light, Material safety data sheet, Sigma Aldrich company limited, March 2004.
- Effect of powder particle size on the strength of Ceramic cores made by injection moulding process, Keyanpour-Rad M, Mehrabi H A, Iranian journal of science and technology, Volume26, p51-54, 2002.
- Modern inorganic chemistry, Liptrot G F, Fourth edition, Bell&Hyman Ltd, p206, 1983.
- Compressive strength and setting temperatures of mixed with various proportions of plaster to stone, Prombonas A, Vlissidis D, Dental school of Athens, Greece, July 1994.
- Ceramic slurry composition and manufacturing method, Miyazaki M, Tanaka S, Takata M, US patent 6790907, September 2004.
- Die material and method of using same, Pearsall, Jeannine A, Aremco products Inc, US patent 4591385, August 1987.
- High temperature characterization of silica base cores for super alloys, Huseby I C, Borom M P, Greskovich C D, G E company, Volume 58, p449-452, 1979.
- Impregnated ceramic core and method of making same, Krug E, US patent 5460854, Certech Incorporated, October1995.
- Material science and engineering an introduction, Callister W D Jr, fifth Edition, pages 663-665, 2000.
- Production of metal casting, Rose K, Doulton company limited, US patent 3473599, January 1965.
- Process of making magnesium oxide cores, Anderson J R, Perron J S, US Patent 3722574, June 1979.
- Ceramic core for investment casting and method for preparation of the same, Kennerknecht S, US patent 5468285, February 1995.
- Leaching of ceramic materials, Mills D, US Patent 5332023, Rolls Royce, London, April 1993
- Moulding, Alexander K, Doulton industrial products ltd, US patent 4629708, May 1984.
- Green cast process, A new ceramic technology for plastic processing, Jarvela P, Jaala A, Advance performance materials, Volume 3, p171-182, April 1996.
- The origin of the term base, Jensen W B, Journal of chemical education, Volume 83, Number.1130, August 2006.
- Core material and method of forming cores, Ferguson T A., US patent 4583581, April 1986.
- Ceramic core and method of making, Keller R J., Haaland, Rodney S, US patent 6578623, June 2003.
- Vitreous fused silica, Gilbert B, Vignot, J M, US patent 5333670, August 1994.
- Fracture toughness of advanced ceramics – Data by dynamic-ceramic Ltd, technical brief, www.azom.com, 19 December 2007.
- Fracture strength for a high strength steel bridge cable wire with a surface crack, Mahmoud K M, Bridge technology consulting, 152-160, 2007.
- Fracture mechanics, Ewalds H.L, Wanhill R.J.H, Arnold E, London, 1984. Water soluble ceramic core for use in die casting, gravity and investment casting of aluminum alloys, Lee Y W, Industrial technology research
Institution, US patent 6024787, June 1998. - Lithium and Lithium Compounds, Ulrich Wietelmann, Bauer RJ, Ullmann's Encyclopedia of Industrial Chemistry, Wiley-CH: Weinheim, 2005.
- Method for treating ceramic cores, Dixon B A, Holt J A, Howmet Research corporation, US patent 805426, March 2005.
- The crystal structure of acetic acid, Jones R.E, Templeton D.H, Act Crystallographia, p 484–87, 1958.
- Material safety data sheet, Acetic, Citric, Nitric acid and Sodium hydroxide, chemical info-box, Wikipedia Webster, wikipedia.com, 2008.
- Chemistry of the elements, Greenwood N. N., Earnshaw A., Second edition., Butterworth-Heinemann, Oxford, 1997.
- Caustic Potash, Oxy.com, Webster, 24 January 2008.
- Method for rapid removal of cores made of Y2O3 from directionally solidified eutectic and super alloy materials, Borom, Marcus P, US patent 4134777, June 1977.
- Apparatus for removing cores from castings, Patrick L. Harold C, Pierson C, McRae M, Howmet Research Corporation, US patent 5915452, April 1999.
- CNC core removal from casting passages, Begg J L, Paul C, US patent 6474348, September 1999.
- Equiaxed lost wax investment casting shell mould characterization, Wardell A T, B Eng Final year project, University of Teesside, p47, Year 96-97.
- Method of casting non-ferrous alloys, Toeniskoetter, Spiwak J, Ashland Oil Inc, US patent 3968828, July 1976.
- Dissolving ceramic materials, Mills D, Rolls Royce, US patent 4569384, November 1986.
- Water base alternative to the use of hydrolysed ethyl silicate in the manufacturing of core and block mould for casting, Robson B, BICTA Conference, 2003.
- Expendable core for casting process, Sang Sial J, Schreck R M, Shah K K, GM Corporation, US patent 5320157, June 1994.