Shuaiying Xi1, Guodong Ma1, Lu Li1,2, Yuanbo Zhang1, Xiangyang Yu1, Yongkun Li3 and Rongfeng Zhou1,2
Published 24 March 2021 • © 2021 The Author(s). Published by IOP Publishing Ltd
Materials Research Express, Volume 8, Number 3
Citation Shuaiying Xi et al 2021 Mater. Res. Express 8 036514
Original content from this work may be used under the terms of the Creative Commons Attribution 4.0 licence. Any further distribution of this work must maintain attribution to the author(s) and the title of the work, journal citation and DOI.
고압 다이캐스팅으로 제작 된 4 wt % TiB 2 / Al-Si-Cu-Zn (T6) 복합 박막 쉘 하우징의 미세 구조 및 기계적 특성
Abstract
The application demand of lightweight high-quality aluminum alloy parts in automotive and aerospace fields is increasingly. In aluminum matrix composites, reinforcing particles can significantly improve the performance of the matrix. In this paper, the microstructures and mechanical properties of die-cast 4 wt%TiB2/Al-9Si-3Cu-0.8Zn composite were systematically analyzed by x-ray diffraction, optical microscope, scanning electron microscopy, energy dispersive spectrometer, transmission electron microscopy and tensile testing. The composite was successfully fabricated from an Al-K2TiF6-KBF4 system by in situ melting technique. The research results show that the average grain sizes of the α-Al phase gradually decreased with the increase of filling distance. And the TiB2 particles were distributed around eutectic Si in irregular polyhedral morphology or nearly circular shape. Meanwhile, the crystal structures of Ti-B compound and long needle shaped nano-sized precipitated were identified and analyzed, and they were found to be TiB2 and Al2Cu phase, respectively. Tensile testing results show that the mechanical properties of die-cast composite clearly increase after T6 heat treatment. The yield strength, ultimate tensile strength and elongation could reach 311 MPa, 379 MPa and 2.8% respectively, with the best injection velocity (1.8 m s−1). The significantly enhancement of mechanical properties of composite after T6 heat treatment was mainly due to the introduction of TiB2 reinforcing phase and the precipitation of Al2Cu precipitate in the aging stage. The results implied that the introduction of TiB2 reinforced particles could improve the mechanical properties of die castings, which has an important guiding role for its practical application.
Korea
자동차 및 항공 우주 분야에서 경량 고품질 알루미늄 합금 부품에 대한 응용 수요가 증가하고 있습니다. 알루미늄 매트릭스 복합재에서 강화 입자는 매트릭스의 성능을 크게 향상시킬 수 있습니다.
본 논문에서는 다이캐스트 4wt%TiB2/Al-9Si-3Cu-0.8Zn 복합체의 미세구조와 기계적 특성을 X선 회절, 광학 현미경, 스캐닝 전자 현미경, 에너지 분산 분광계, 전송 전자 현미경 및 인장 시험에 의해 체계적으로 분석하였습니다. 복합 재료는 현장 용해 기술에 의해 Al-K2TiF6-KBF4 시스템에서 성공적으로 제작되었습니다.
연구 결과에 따르면 α-Al 단계의 평균 곡물 크기는 충전 거리가 증가함에 따라 점차 감소했습니다. 그리고 TiB2 입자들은 불규칙한 다면체 형태학 또는 거의 원형에 가까운 형태로 유전자 Si 주변에 분포했습니다. 한편, Ti-B 화합물의 결정 구조와 긴 바늘 모양의 나노 크기의 침전물이 확인되고 분석되었으며, 각각 TiB2상 및 Al2Cu상인 것으로 확인되었습니다. 인장 테스트 결과, 다이캐스트 합성물의 기계적 특성은 T6 열처리 후 확실히 증가한다는 것을 알 수 있습니다.
항복 강도, 최종 인장 강도 및 연장은 최고 분사 속도(1.8 ms-1)로 각각 311 MPa, 379 MPa 및 2.8%에 이를 수 있습니다. T6 열처리 후 합성물의 기계적 특성이 크게 향상된 것은 주로 TiB2 강화 단계가 도입되고 노화 단계에서 Al2C 침전물이 침전했기 때문입니다. 결과는 TiB2 강화 입자의 도입으로 다이 주물의 기계적 특성이 개선될 수 있다는 것을 시사했습니다. 다이 주물의 기계적 특성은 실제 적용에서 중요한 지침 역할을 합니다.
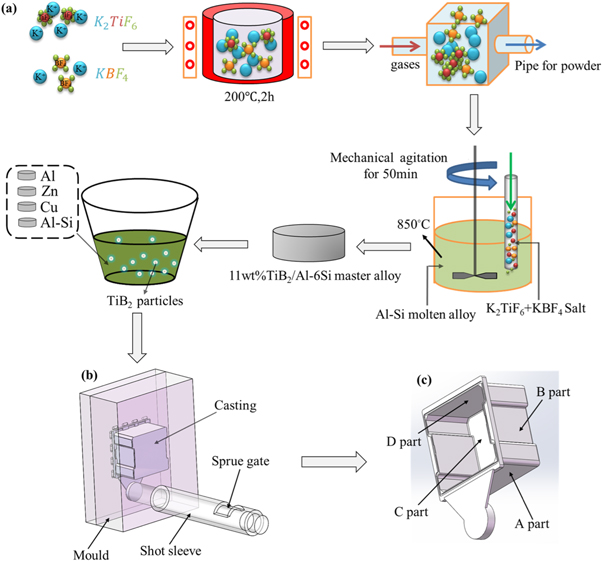
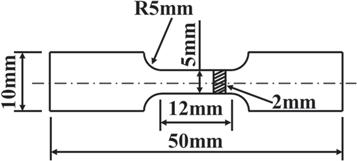
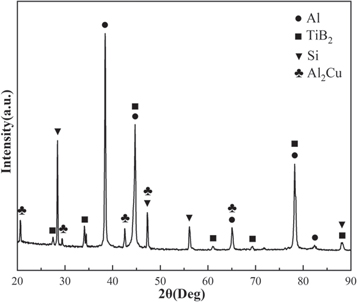
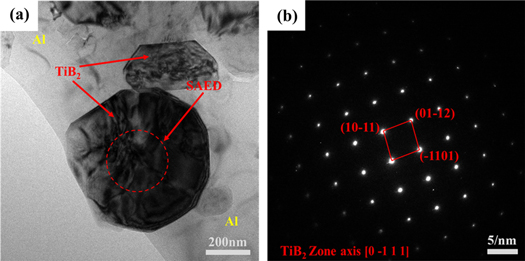
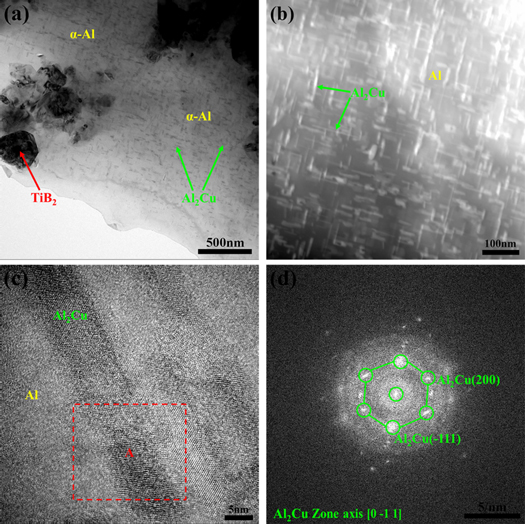
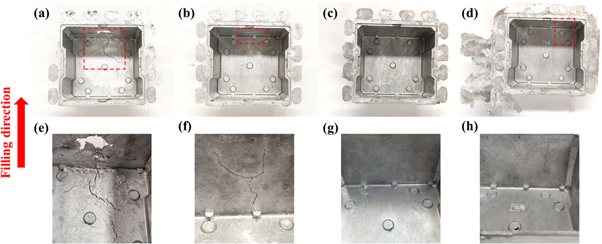
Reference
[1]
Dong X, Huang X, Liu L, He L and Li P 2016 A liquid aluminum alloy electromagnetic transport process for high pressure die casting J. Mater. Process. Technol. 234 217–27
CrossrefGoogle Scholar
[2]
Choukri K M, Nabil K A I and Said A 2019 Effects of shot sleeve filling in HPDC machine Multidiscipline Modeling in Materials and Structures 15 1255–73
CrossrefGoogle Scholar
[3]
Yan P, Mao W, Fan J, Wang B and Liu Y 2020 Microstructural evolution, segregation and fracture behavior of A390 alloy prepared by combined Rheo-HPDC processing and Sr-modifier J. Alloys Compd. 835 155297
CrossrefGoogle Scholar
[4]
Outmani I, Fouilland-Paille L, Isselin J and Mansori M E 2017 Effect of Si, Cu and processing parameters on Al-Si-Cu HPDC castings J. Mater. Process. Technol. 249 559–69
CrossrefGoogle Scholar
[5]
Qi M, Li J and Kang Y 2019 Correlation between segregation behavior and wall thickness in a rheological high pressure die-casting AC46000 aluminum alloy Journal of Materials Research and Technology 8 3565–79
CrossrefGoogle Scholar
[6]
Cecchel S, Ferrario D, Panvini A and Cornacchia G 2018 Lightweight of a cross beam for commercial vehicles: development, testing and validation Mater. Des. 149 122–34
CrossrefGoogle Scholar
[7]
Saha S, Tareq S H and Galid R H 2016 Effect of overageing conditions on microstructure and mechanical properties in Al–Si–Mg Alloy Journal of Material Sciences & Engineering 5 321–5
CrossrefGoogle Scholar
[8]
Alam M T, Arif S, Ansari A H and Alam M N 2019 Optimization of wear behaviour using Taguchi and ANN of fabricated aluminium matrix nanocomposites by two-step stir casting Mater. Res. Express 6 065002
IOPscienceGoogle Scholar
[9]
Pramod S L, Prasada Rao A K, Murty B S and Bakshi S R 2015 Effect of Sc addition on the microstructure and wear properties of A356 alloy and A356-TiB2 in situ composite Mater. Des. 78 85–94
CrossrefGoogle Scholar
[10]
Bauri R, Yadav D, Shyam Kumar C N and Balaji B 2015 Tungsten particle reinforced Al 5083 composite with high strength and ductility Materials Science and Engineering: A 620 67–75
CrossrefGoogle Scholar
[11]
Tjong S C and Ma Z Y 2000 Microstructural and mechanical characteristics of in situ metal matrix composites Materials Science & Engineering 29 49–113
CrossrefGoogle Scholar
[12]
Alam M T, Arif S and Ansari A H 2018 Wear behaviour and morphology of stir cast aluminium/SiC nanocomposites Mater. Res. Express 5 045008
IOPscienceGoogle Scholar
[13]
Su J, Li Y, Duan M-G, Liu S and Liu K 2018 Investigation on particle strengthening effect in in situ TiB2/2024 composite by nanoindentation test Materials Science and Engineering: A 727 29–37
CrossrefGoogle Scholar
[14]
Alam M T, Ansari A H, Arif S and Alam M N 2017 Mechanical properties and morphology of aluminium metal matrix nanocomposites-stir cast products Advances in Materials and Processing Technologies 4 600–15
CrossrefGoogle Scholar
[15]
Tian K, Zhao Y, Jiao L, Zhang S, Zhang Z and Wu X 2014 Effects of in situ generated ZrB2 nano-particles on microstructure and tensile properties of 2024Al matrix composites J. Alloys Compd. 594 1–6
CrossrefGoogle Scholar
[16]
Han Q, Geng Y, Setchi R, Lacan F, Gu D and Evans S L 2017 Macro and nanoscale wear behaviour of Al-Al2O3 nanocomposites fabricated by selective laser melting Composites Part B: Engineering 127 26–35
CrossrefGoogle Scholar
[17]
Yuan P, Gu D and Dai D 2015 Particulate migration behavior and its mechanism during selective laser melting of TiC reinforced Al matrix nanocomposites Mater. Des. 82 46–55
CrossrefGoogle Scholar
[18]
Gowri Shankar M C, Jayashree P K, Shettya R, Kinia A and Sharma S S 2013 Individual and combined effect of reinforcements on stir cast aluminium metal matrix composites-a review International Journal of Current Engineering and Technology 3 922–34
Google Scholar
[19]
He N, Zhang J, Jin Y, Han F, Zhang X and Chen G 2019 Preparation, microstructures and mechanical properties of in situ TiB2/Al composites by mechanical stirring and subsequent ultrasonic treatment Mater. Res. Express 6 116553
IOPscienceGoogle Scholar
[20]
Gao Q, Wu S, LÜ S, Duan X and An P 2016 Preparation of in situ 5 vol% TiB2 particulate reinforced Al-4.5Cu alloy matrix composites assisted by improved mechanical stirring process Mater. Des. 94 79–86
CrossrefGoogle Scholar
[21]
Wu L, Zhou C, Li X, Ma N and Wang H 2018 Effects of TiB2 particles on artificial aging response of high-Li-content TiB2/Al-Li-Cu composite J. Alloys Compd. 749 189–96
CrossrefGoogle Scholar
[22]
Mozammil S, Karloopia J, Verma R and Jha P K 2019 Effect of varying TiB2 reinforcement and its ageing behaviour on tensile and hardness properties of in situ Al-4.5%Cu-xTiB2 composite J. Alloys Compd. 793 454–66
CrossrefGoogle Scholar
[23]
Zeren M, Karakulak E and Gümüş S 2011 Influence of Cu addition on microstructure and hardness of near-eutectic Al-Si-xCu-alloys Transactions of Nonferrous Metals Society of China 21 1698–702
CrossrefGoogle Scholar
[24]
Zhang S L, Shi X X, Zhao Y T, Zhang B R, Liang Z P, Yin H S, Dou B Y, Zhang Q and Wang C X 2016 Preparation, microstructures and mechanical properties of in situ (TiB2 + ZrB2)/AlSi9Cu3 composites J. Alloys Compd. 673 349–57
CrossrefGoogle Scholar
[25]
Wen X, Wang Q, Mu Q, Kang N, Sui S, Yang H, Lin X and Huang W 2019 Laser solid forming additive manufacturing TiB2 reinforced 2024Al composite: microstructure and mechanical properties Materials Science & Engineering: A 745 319–25
CrossrefGoogle Scholar
[26]
Dong X, Youssef H, Zhu X, Zhang Y, Wang S and Ji S 2021 High as-cast strength die-cast AlSi9Cu2Mg alloy prepared by nanoparticle strengthening with industrially acceptable ductility J. Alloys Compd. 852 156873
CrossrefGoogle Scholar
[27]
Xiao Y, Li L, Wang Q, Li Y, Zhou R and Jiang Y 2020 Microstructural characteristics and evolution of in situ xTiB2/Al-20%Si alloy Mater. Res. Express 6 1265i1
IOPscienceGoogle Scholar
[28]
Leskovar B, Samardžija Z, Koblar M, Naglič I and Markoli B 2019 Development of an Al-Mn-Si-based alloy with an improved quasicrystalline-forming ability JOM 72 1533–9
CrossrefGoogle Scholar
[29]
Wang R, Guo W, Wang J, Yuan K, Liu L, Li P and Li Y 2020 Effects of stress state, strain rate, and temperature on fracture behavior of in situ TiB2/2024 Al composite Mech. Mater. 151 103641
CrossrefGoogle Scholar
[30]
Amirkhanlou S, Ji S, Zhang Y, Watson D and Fan Z 2017 High modulus Al-Si-Mg-Cu/Mg2Si-TiB2 hybrid nanocomposite: microstructural characteristics and micromechanics-based analysis J. Alloys Compd. 694 313–24
CrossrefGoogle Scholar
[31]
Du R, Gao Q, Wu S, Lü S and Zhou X 2018 Influence of TiB2 particles on aging behavior of in situ TiB2/Al-4.5Cu composites Materials Science & Engineering: A 721 244–50
CrossrefGoogle Scholar