. Jelínek, E. Adámková*
Department of Metallurgy and Foundry Engineering, VŠB-Technical University of Ostrava,
- listopadu 2172/15, 708 33 Ostrava – Poruba, Czech Republic
*Corresponding author. E-mail address: eliska.adamkova@vsb.cz
Received 04.03.2014; accepted in revised form 30.03.2014
Abstract
Development of salt cores prepared by high-pressure squeezing and shooting with inorganic binders has shown a high potential of the given technology even for high-pressure casting of castings. Strength, surface quality of achieved castings, and solubility in water become a decisive criterion. The shape and quality of grain surface particularly of NaCl – cooking salts that can be well applied without anticaking additives has shown to be an important criterion. Thus the salt cores technology can cover increasingly growing demands for casting complexity especially for the automobile industry.
Keywords: Innovative foundry technologies and materials, Product development, Salt cores, High-pressure die casting, Squeezinginjection
Introduction
Classic metal cores used for high-pressure casting of castings cannot entirely cover increasingly growing demands for shape complexity of castings especially for automobile industry [1, 2]. Therefore the interest in development of soluble cores (Verlorene Kerne, Lost Cores) that would also enable at the same time the closed technological cycle is growing. This prerequisite is met by cores from inorganic salts soluble in water. But the application of them is limited above all by strength properties and both under low temperatures (primary strength) and under increased temperatures too. On the other hand it is also limited by casting conditions – injecting of metal into the mould (the rate in ingates, post-pressure, laminar filling, gating system). According to some authors the salt cores are suitable for lower filling rates (under 35 m/s) with limited post-pressure, i.e. ideal e.g. for the „rheocasting“ technology [3]. The contribution deal with development of salt cores by way of high-pressure squeezing and shooting with use of alkali silicate binders and their applications on test high-pressure cast castings.
New processes of cores manufacture
- Sand cores – made by the classic PUR Cold – Box technology (the polyurethanes binder) or Warm – Box (alkalisilicates). Blank cast holes are geometrically accurate but the cores are wrongly collapsible (annealing is necessary).
Against penetration it is necessary to optimize the granulometry of base sands and to use protective coatings. Sometimes it is recommended to dip the cores in the binder (resin, water glass) [3]. - Plastic cores (polyoxmethylen) – are made by working from blocks. Removing is done by burning the core residues. Cores from low-melting metals, e.g. Zn-alloys (ZnAl4Cu1) [3]. They must be melt out by additional annealing of castings. But the surface is of a high quality corresponding to that one obtained with permanent mould casting.
- Cores from inorganic salts
- Hollow cores combined with a metal tube [4]
- Full cores
For high-pressure casting the highest potential represent the salt cores. Their use for gravity and low-pressure casting is known for a longer time but their application for demanding conditions of high-pressure casting necessitates next research. Three technologies of sand cores preparation are developed in substance (Fig. 1). Each of them has its own advantages and disadvantages too.
Our research is aimed at squeezing of crystalline salts and shooting with use of a binder (alkali silicates).
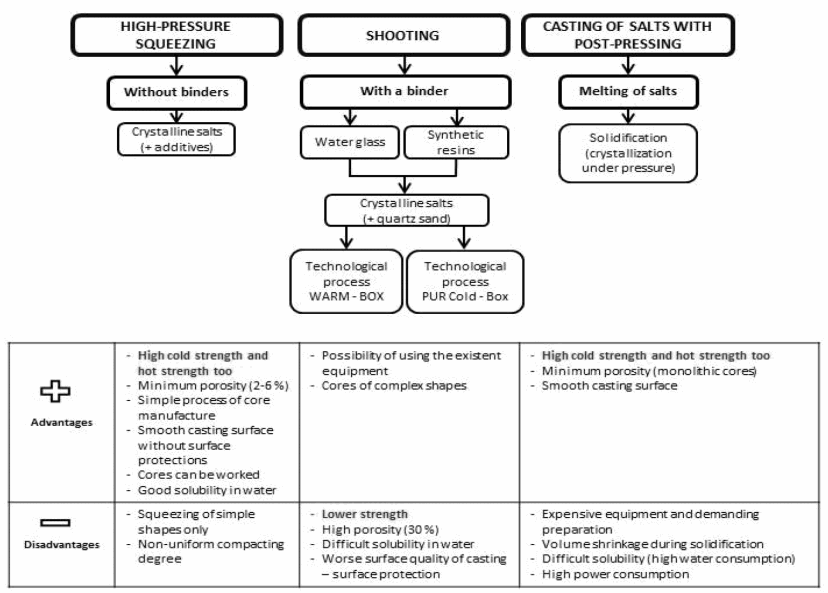
Salt cores made by shooting and squeezing
NaCl and KCl are included among the most frequently used salts. Some deficiencies are solved by mixtures of salts with minority share of carbonates, sulphates, and phosphates of alkaline metals. Our first experiments resulted from NaCl and KCl of chemical purity. In spite of the fact that physical-chemical properties of both salts are not substantially different the KCl in all cases shows considerably higher strengths both under low temperatures and under high temperatures (up to 650 °C) too what used to be explained in particular by different grain angularity and shape [5]. Cardinal importance of the shape and quality of the grain surface has been proved in case of NaCl (cooking salt) of different origin and provenance [6]. Composition of cooking salt meets both hygienic (iodination, fluorination) and technical requirements. In some salts the additives of anticaking matters are found, and namely K2CO3, CaCO3, MgCO3, SiO2, K4[Fe(CN)6]·3H2O. For determination an importance of the grain shape 6 kinds of NaCl of fraction 0.06 – 1.0 mm were evaluated as follows:
1 – Rock salt with I, F 4 – Alpine salt with I, F
2 – Rock salt with I 5 – Sea salt
3 – Alpine salt with I 6 – NaCl, chemically pure, standard
and namely the grains of the shape of crushed material (1, 2), of regular cube (3, 4) up to oval grains (5) and the dipyramidal form (6). While the high-pressure squeezed cores (104 MPa) were made from crystalline salts only, with the shooting method the cores were made with the alkali silicate binder and they were hot hardened (190 °C). Squeezed cores achieve by 2 – 3 times higher bending strengths than shot cores (Fig. 2). The core porosity correlates with it too and it ranges for the squeezed cores under 6 % while for the shot ones it is 35 – 40 %. On the one hand the porosity helps in metal penetration (deterioration of surface quality) but on the other it accelerates dissolving. The lowest strength has shown the Alpine salts recrystallized to grains of a cubic form. But the surface analysis has proved the presence of anticaking matters (MgCO3, CaCO3, Fig. 3) that prevent from recrystallization of etched grain surfaces after compacting the cores. The highest strengths were achieved (particularly in case of squeezed ones) in case of oval grains of sea salt and the grains of the dipyramidal shape that are most similar to the shape of SiO2 grains.
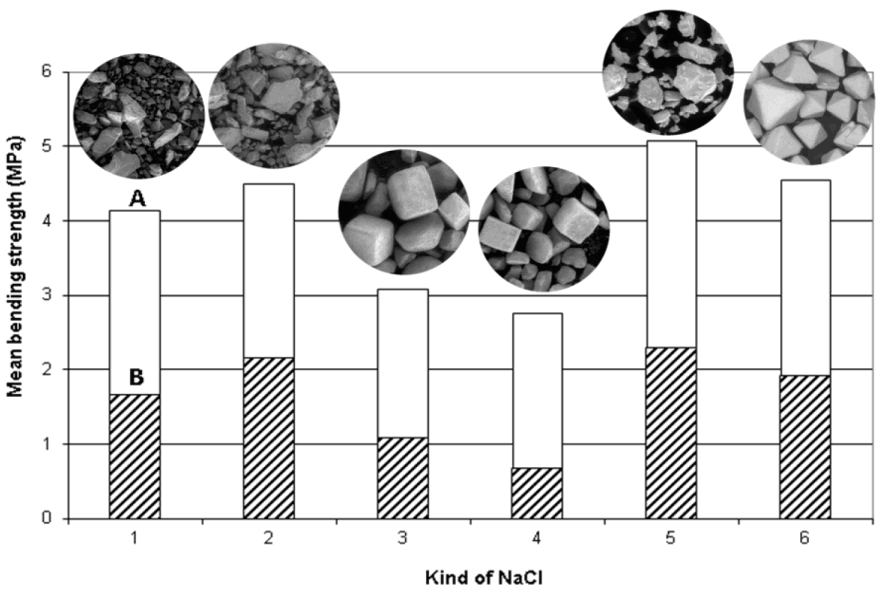
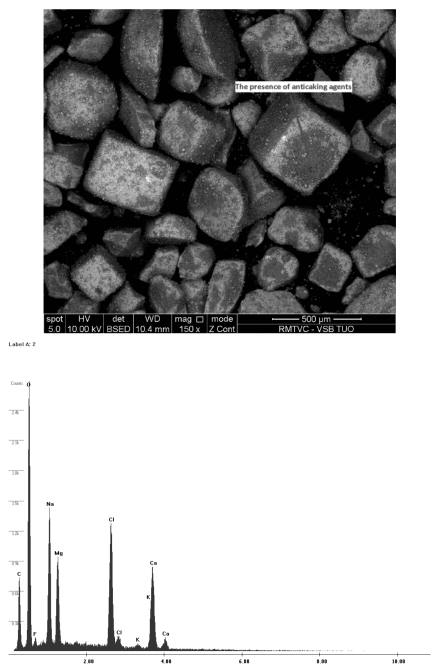
Further growth of cold and heat strengths is possible by applying of composite salts resulting from the presumption that it can be achieved by disturbing of long dislocation lines with finely dispersed particles of high heat resistance present in the salt matrix. The basis is formed by KCl and NaCl crystalline salts and ZrSiO4, Al2O3, SiO2, Fe2O3 of defined concentration and granulometry were used as composites. Application of them has shown as follows:
- the growth of primary strength of cores squeezed with force up to 200 kN in correlation with the decrease of porosity
- additives increase the strength under high temperatures (650 °C) of cores squeezed even with low pressures (52 MPa)
- maximum bending strength was achieved with Al2O3 (> 9 MPa) what is ca a treble of strength of the PUR Cold – Box cores
- residual strength after the exposure of 650 °C/ 1 h is by about 10 – 20 % higher that the primary strength
- quality of blank cast holes substantially increased (Ra < 3 – 5 µm)
- high kinetics of dissolution of cores in water is kept Cores made by high-pressure squeezing are characteristic with minimum porosity, high primary strength [7] but also with non uniformity of compaction in the squeezing height and especially with high residual stress that resulted in formation of shrinkage cracks.
Those are already formed during disassembling of the core box or during the proper pouring of castings.
One of solution ways consisted in annealing of cores, and namely to 180; 350; and 550/650 °C/2 h and residual bending strength, the abrasive wear and the kinetics of dissolution in water were studied at the same time.
It has been shown (Fig. 4) that annealing of cores from mixtures 1 and 2 above 350 °C has no considerable influence on residual strength when squeezing with force 100 kN while extreme squeezing (200 kN) further on increases the bending strength when after annealing (650 °C) it exceeds 9 MPa (mixture 3).
From temperature of 175 °C the mixtures 2 and 3 exceed the required bending strength limit for salt cores of 6.5 MPa [8].
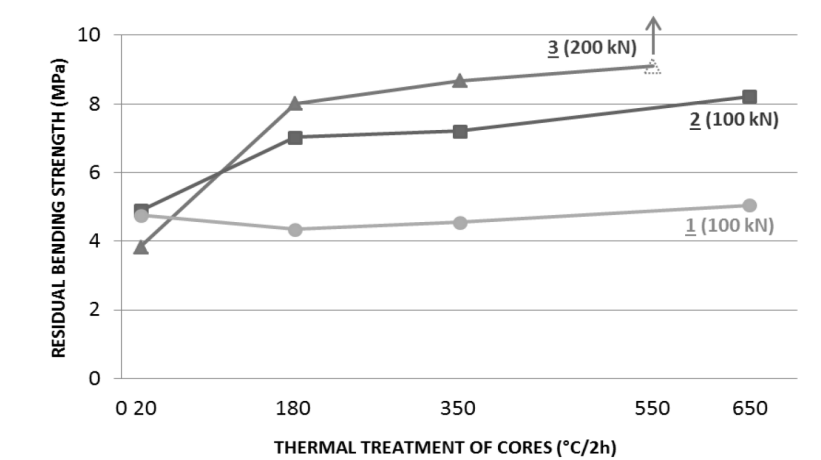
With thermal exposure of the cores from mixtures 2 and 3 the recrystallization processes of the salt system were completed. In correlation with the growth of residual strength the abrasive wear of cores considerably decreases (Fig. 5).
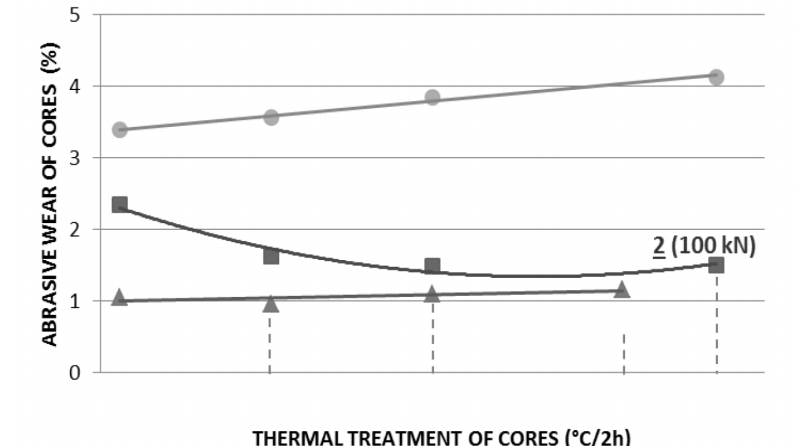
Real porosity of cores decreased below 6.2 % with mean diameter of pores of 0.0618 µm what is a precondition to preventing the metal penetration and obtaining the perfect smoothness of the blank cast hole. On the other hand this fact can hamper the whole kinetics of cores dissolution.
An important finding was a fact that annealing temperature has no negative influence on the kinetics of cores dissolution in water. On the contrary the longest dissolution times had the cores solidifying in the open air (48 h/20 °C) and after annealing to 180 °C (Fig. 6.). All the cores were dissolved within 32 min. in 20 °C still water.
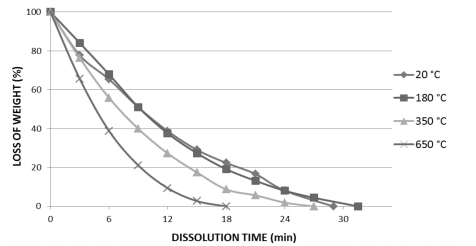
High quality salt cores without shrinkage cracks made by high-pressure squeezing were manufactured under conditions as follows:
- by decreasing the pressing force to 100 kN
- by a shaped die head
- by thermal treatment of cores (annealing 350 °C)
- by protective spraying of the core box (based on BN)
- by carbonaceous additive for decreasing of internal friction
of composite crystalline salts Results of these treatments on salt cores were checked on test castings.
Test castings
Salt cores were checked in operating conditions of the joint stock company of KOVOLIS HEDVIKOV, a.s. on a casting machine CLH 400 with compacting pressure in a chamber. The cast AlSi9Cu3(Fe) alloy was injected with rate of 16 and 35 m/s in ingates and the position of the test core towards the runner can be changed by 0° (against the runner), 90° and 180°. With use of cores from composite squeezed salts (100 kN) the high smoothness of blank cast holes (Ra < 3 – 4 µm), geometrical accuracy, and scavenging with freely flowing water (38 – 40 °C) within 7 – 8 min. were achieved (Fig. 7).
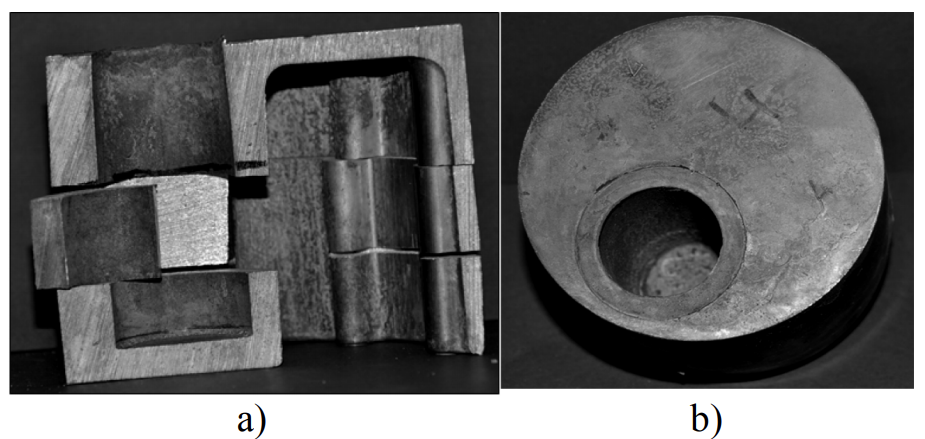
Conclusion
Salt water soluble squeezed cores from composite mixtures represent an extensive potential also for the technology of high pressure cast castings. In case of shot cores from cooking salts of a higher shape complexity with the presence of alkali silicate binders the further development is required especially for surface protection with the aim of achieving the higher smoothness of blank cast holes.
Acknowledgement
The research was realized with financial support of the Technological Agency of the Czech Republic in the Alfa TA 020 11314 programme.
Reference
References
[1] Brůna, M., Sládek, A. & Kucharčík, L. (2012). Formation of porosity in Al-Si alloys. Archives of Foundry Engineering. 12(1), 5-8. DOI: 10.2478/v10266-012-0001-5.
[2] Lichý, P., Bednářová, V. & Elbel, T. (2012). Casting routes for porous metals production. Archives of Foundry Engineering. 12(1), 71-74. DOI: 10.2478/v10266-012-0014-0.
[3] Michels, H., Bűnck, M. & Bűhrig – Polaczek, A. (2010). Suitability of lost cores in rheocasting process. Transactions of Nonferrous Metals Society of China. 20, 948-953. DOI: 10.1016/S1003-6326(10)60612-7.
[4] Kallien, L., Bőhnlein, Ch., Dworak, A. & Műller, B. (2013). Ergebnisse aus dem Forschungsprojekt 3-D-Freiform – medienführende Kanäle im Druckguss. Giesserei Praxis. 100(12), 36-43.
[5] Jelínek, P., Mikšovský, F., Beňo, J. & Adámková, E. (2013). Development of Foundry Cores Based on Inorganic Salts. Materials and technology. 47(6), 689-693. ISSN: 1580-2949, 1580-3414.
[6] Adámková, E., Jelínek, P., & Študentová, S. (2013). Application of cooking salts in manufacture of water soluble cores for high pressure die casting (Aplikace kuchyňských solí při výrobě vodou rozpustných jader odlitků tlakového lití). Materials and technology. 61(11-12), 689-693. ISSN: 1580-2949, 1580-3414.
[7] Jelínek, P., Mikšovský, F., & Adámková, E. (2012). Influencing the strength characteristics of salt cores soluble in water (Ovlivňování pevnostních charakteristik solných ve vodě rozpustných jader). Slévárenství. 60(3-4), 85-89. ISSN: 0037-6825.
[8] Fuchs, B., Eibisch, H. & Körner, C. (2013). Core viability simulation for salt core technology in high – pressure die casting. International Journal of Metalcasting. 7(3), 39-45. ISSN: 1939-5981.