This introduction paper is based on the paper "Friction Stir Processing: A Thermomechanical Processing Tool for High Pressure Die Cast Al-Alloys for Vehicle Light-weighting" published by "Manufacturing Letters".
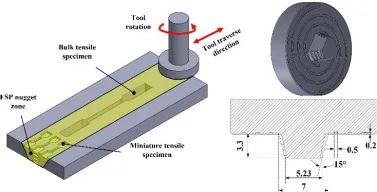
1. Overview:
- Title: Friction Stir Processing: A Thermomechanical Processing Tool for High Pressure Die Cast Al-Alloys for Vehicle Light-weighting
- Author: Avik Samanta, Hrishikesh Das, Glenn J. Grant, Saumyadeep Jana
- Year of publication: 2024
- Journal/academic society of publication: Manufacturing Letters
- Keywords: Friction stir processing; High pressure die casting; Tensile properties; Fatigue; Tear toughness
2. Abstract:
This study uses friction stir processing (FSP) for thermomechanical processing of high-pressure die-casting (HPDC) to modify microstructure and improve mechanical properties. FSP is carried out on two different HPDC aluminum alloys: (a) general-purpose, high-iron, HPDC A380 alloy and (b) premium quality, low-iron HPDC Aural-5 alloy in thin wall, flat plate geometry. Subsequent mechanical testing shows ~30% and ~65% enhancement in yield strength and tensile ductility. In addition, FSP leads to ~10 times improvement in fatigue life for A380 alloy and ~70% improvement in fracture toughness for Aural-5 alloy. These findings emphasize the capability of FSP to modify the microstructure of HPDC Al-alloys-based structural components so that they can demonstrate a good combination of strength, ductility, fracture toughness, and high fatigue properties for long-term durability and reliability.
3. Introduction:
The increasing demand for zero carbon emissions drives automotive original equipment manufacturers (OEMs) to seek cost-effective solutions for light-duty (LD) vehicle weight reduction. Replacing heavy structural steel assemblies with lightweight aluminum structural castings is a key strategy. High pressure die-cast (HPDC) aluminum castings offer advantages in design, weight reduction, and quality for critical load-bearing body structures in automotive designs. These include shock towers, pillars, and floor rails. HPDC Al castings also enable intricate structural profiles and effective part unitizing. As electric vehicle technology advances, HPDC aluminum castings become crucial for lightweight enclosures for batteries and powertrain components.
A significant challenge in the Al die-casting industry is balancing die-life extension and ductility of the final casting. Conventional approaches often involve modifying material chemistry. For general-purpose die-casting, iron (Fe) and manganese (Mn) are used to reduce die wear, although high Fe content can reduce ductility and fatigue life. Silicon (Si) is added to improve fluidity, feeding rate, and hot tear resistance. Copper (Cu), zinc (Zn), and magnesium (Mg) contribute to strengthening HPDC alloys. However, HPDC Al alloys often exhibit complex intermetallic compounds, such as needle-shaped β-FeSiAl (FeSiAl5) and polyhedral α-FeSiAl (Al15(MnFe)3Si2), which can detrimentally affect mechanical characteristics, especially ductility. Acicular silicon and second-phase particulates, inherent dendritic microstructure, and gas/shrinkage porosity in HPDC Al alloys further limit their use in structural components.
4. Summary of the study:
Background of the research topic:
HPDC aluminum castings, despite their advantages, suffer from inherent microstructural defects and material chemistry limitations that compromise mechanical properties like ductility, fatigue life, and fracture toughness. These limitations hinder their broader application in vehicle structural components, particularly in the context of increasing demands for lightweighting and durability in the automotive industry.
Status of previous research:
Previous research efforts have focused on modifying alloy chemistry and HPDC processes to mitigate detrimental features. Vacuum-assisted HPDC reduces gas and shrinkage porosity. Premium low-Fe HPDC Al-alloys, such as Silafont, Castasil, and Aural, have been developed to improve ductility by eliminating detrimental beta phases. Strontium (Sr) addition is used to modify acicular silicon into a finer, fibrous structure, enhancing strength and ductility. Sr-modified alloys, like Aural-5, have seen successful structural applications. However, challenges remain, including shrinkage porosity, dendritic microstructure, shear-band formation, externally solidified crystals (ESCs), and second-phase particulates.
Purpose of the study:
The study aims to investigate friction stir processing (FSP) as a thermomechanical post-processing tool to modify the microstructure of thin-wall HPDC Al-alloys and enhance their mechanical properties. This approach offers an alternative to costly alloy composition and HPDC process optimization by directly addressing microstructural defects and limitations responsible for reduced mechanical performance.
Core study:
The core study involves applying FSP to two different HPDC aluminum alloys: (i) high-iron A380 and (ii) low-iron Aural-5, both in thin-wall, flat plate geometries. The research assesses the impact of FSP on microstructure evolution and mechanical properties, including tensile properties, fatigue life, and tear toughness. Mechanical performance is evaluated through coupon-level testing to demonstrate the effectiveness of FSP in improving the overall performance of HPDC Al-alloys for structural applications.
5. Research Methodology
Research Design:
The research employs an experimental design involving FSP of HPDC A380 and Aural-5 alloy plates. The study compares the microstructure and mechanical properties of processed (FSPed) and unprocessed (HPDC) materials. Two HPDC Al-alloys were selected: (i) high-Fe containing A380 and (ii) low-Fe containing Aural-5. FSP trials were conducted on flat plate geometries (3.5 mm thick A380 and 2.5 mm thick Aural-5).
Data Collection and Analysis Methods:
- Microstructure Characterization: Optical Microscopy (OM) and Scanning Electron Microscopy (SEM) were used to examine the microstructure. ImageJ software was used for quantitative analysis of microstructural features, including equivalent circular diameter (ECD) and aspect ratio of phases. SEM-EDS analysis was used for elemental mapping.
- Tensile Testing: Full-thickness subsize ASTM E8 specimens and miniature tensile specimens were used to evaluate tensile properties (Yield Strength (YS), Ultimate Tensile Strength (UTS), and % Elongation).
- Fatigue Testing: High cycle bending fatigue tests were performed on full-thickness rectangular specimens under a 4-point bending configuration at a stress ratio of R=0.1.
- Tear Toughness Testing: Conventional tear tests were conducted according to ASTM standard B871-01 using rectangular specimens with a V-notch to assess tear toughness. Numerical integration was used to calculate unit total energy from force-displacement curves.
Research Topics and Scope:
The research focuses on:
- Investigating the effect of FSP on microstructure modification in HPDC A380 and Aural-5 alloys.
- Evaluating the improvement in tensile properties (yield strength, tensile ductility) after FSP.
- Assessing the enhancement in fatigue life of HPDC A380 alloy due to FSP.
- Determining the impact of FSP on tear toughness of HPDC Aural-5 alloy.
- Comparing the mechanical performance of FSPed material to that of as-cast HPDC material in different regions (die-wall vs. mid-wall).
6. Key Results:
Key Results:
- Microstructure Evolution: FSP effectively eliminated casting porosity and refined the dendritic microstructure in both A380 and Aural-5 alloys, transforming it into a wrought microstructure with uniformly distributed fragmented silicon and second-phase particles. The size and aspect ratio of silicon particles and second-phase particles were significantly reduced after FSP.
- Tensile Property Improvement: FSP led to significant enhancements in tensile properties. Yield strength (YS) improved by ~25% for A380 and ~30% for Aural-5. Tensile ductility (% Elongation) increased by ~65% for A380 and ~35% for Aural-5. Ultimate Tensile Strength (UTS) remained largely unchanged.
- Fatigue Life Enhancement: FSP significantly improved the high cycle fatigue life of A380 alloy in bending mode, showing a ~5-15 times improvement at higher stress levels and more than 15-fold improvement at lower stress levels compared to HPDC A380.
- Tear Toughness Improvement: FSP resulted in a ~70% enhancement in tear toughness for Aural-5 alloy, indicating increased resistance to crack initiation and propagation.
Figure Name List:
- Fig. 1. Schematic of FSP experimental setting with tool shoulder and pin geometry and position of miniature and bulk E8 tensile specimens with respect to FSP nugget zone.
- Fig. 2. Low-magnification microstructural overview of (a) HPDC A380; (b) FSPed A380; (c) HPDC Aural-5; (b) FSPed Aural-5.
- Fig. 3. Comparison of SEM microstructure of HPDC and FSPed material in locations (A) to (E) in Figure 2.
- Fig. 4. EDS Analysis of HPDC A380 alloy at (a) die-wall, (b) mid-wall, and (c) FSPed mid-wall, and HPDC Aural-5 alloy at (d) mid-wall and (e) FSPed mid-wall.
- Fig. 5. Comparison of engineering stress vs. percent elongation of bulk tensile specimen.
- Fig. 6. Combination of yield strength and % elongation to failure for HPDC and FSPed (a) A380 alloy and (b) Aural-5 alloy
- Fig. 7. Fatigue life improvement of HPDC A380 alloy after FSP: (a) location of fatigue specimen with the nugget zone, and (b) comparison of no. of cycles to failure for different stress levels
- Fig. 8. Tear toughness test for Aural-5: (a) Sample geometry; and Comparison of (b) the force-displacement curve and (c) unit total energy.
7. Conclusion:
This study demonstrates the effectiveness of Friction Stir Processing (FSP) as a thermomechanical tool to enhance the mechanical properties of HPDC aluminum alloys, irrespective of alloy chemistry (high-Fe A380 and low-Fe Aural-5). FSP-driven microstructure modification led to improved tensile ductility (~65% for A380, ~35% for Aural-5) and yield strength (~25% for A380, ~30% for Aural-5). Significant improvements were also observed in high cycle fatigue life for A380 (~5 to >15 times) and tear toughness for Aural-5 (~70%). The enhanced mechanical properties are attributed to the elimination of casting defects like porosity and the refinement of the dendritic microstructure into a homogeneous wrought microstructure with fragmented and uniformly distributed phases.
8. References:
- [1] COSMO International. Aluminum High Pressure Die Casting. 2014.
- [2] Sigworth GK, Donahue RJ. The Metallurgy of Aluminum Alloys for Structural High-Pressure Die Castings. International Journal of Metalcasting 2021;15:1031-46. https://doi.org/10.1007/s40962-020-00535-х.
- [3] Hartlieb M. Aluminum alloys for structural die casting. Die Casting Engineer, 2013, p. 40-3.
- [4] Outmani I, Fouilland-Paille L, Isselin J, el Mansori M. Effect of Si, Cu and processing parameters on Al-Si-Cu HPDC castings. J Mater Process Technol 2017;249:559-69. https://doi.org/10.1016/j.jmatprotec.2017.06.043.
- [5] Niu XP, Hu BH, Pinwill I, Li H. Vacuum assisted high pressure die casting of aluminium alloys. J Mater Process Technol 2000;105:119-27. https://doi.org/10.1016/S0924-0136(00)00545-8.
- [6] Rowe J, editor. Advanced Materials in Automotive Engineering. Woodhead Publishing; 2012.
- [7] Sigworth GK. The Modification of Al-Si Casting Alloys: Important Practical and Theoretical Aspects. International Journal of Metalcasting 2008;2:19-40. https://doi.org/10.1007/BF03355425.
- [8] Hegde S, Prabhu KN. Modification of eutectic silicon in Al-Si alloys. J Mater Sci 2008;43:3009-27. https://doi.org/10.1007/s10853-008-2505-5.
- [9] Timpel M, Wanderka N, Schlesiger R, Yamamoto T, Lazarev N, Isheim D, et al. The role of strontium in modifying aluminium-silicon alloys. Acta Mater 2012;60:3920-8. https://doi.org/10.1016/j.actamat.2012.03.031.
- [10] Shabestari SG, Keshavarz M, Hejazi MM. Effect of strontium on the kinetics of formation and segregation of intermetallic compounds in A380 aluminum alloy. J Alloys Compd 2009;477:892–9. https://doi.org/10.1016/j.jallcom.2008.11.037.
- [11] Ganesh MRS, Reghunath N, J.Levin M, Prasad A, Doondi S, Shankar K v. Strontium in Al-Si-Mg Alloy: A Review. Metals and Materials International 2022;28. https://doi.org/10.1007/s12540-021-01054-y.
- [12] Shih TS, Shih FS. Effects of silicon, magnesium and strontium content on the qualities of Al-Si-Mg alloys. International Journal of Cast Metals Research 1998;10:273-82. https://doi.org/10.1080/13640461.1998.11819243.
- [13] Beals R, Niu X, Brown Z. Development of Advanced Aluminum Alloy for Structural Castings. In: Eskin D, editor. Light Metals 2022, Cham: Springer International Publishing; 2022, p. 73–82. https://doi.org/10.1007/978-3-030-92529-1_10.
- [14] Beals R, Conklin J, Skszek T, Zaluzec M, Wagner D. Aluminum High Pressure Vacuum Die Casting Applications for the Multi Material Lightweight Vehicle Program (MMLV) Body Structure. In: Hyland M, editor. Light Metals 2015, Cham: Springer International Publishing; 2015, p. 215-21. https://doi.org/10.1007/978-3-319-48248-4_38.
- [15] Sharma SR, Ma ZY, Mishra RS. Effect of friction stir processing on fatigue behavior of A356 alloy. Scr Mater 2004;51:237-41. https://doi.org/10.1016/j.scriptamat.2004.04.014.
- [16] Tsai FY, Kao PW. Improvement of mechanical properties of a cast Al-Si base alloy by friction stir processing. Mater Lett 2012;80:40-2. https://doi.org/10.1016/j.matlet.2012.04.073.
- [17] Freeney TA, Mishra RS. Effect of friction stir processing on microstructure and mechanical properties of a cast-magnesium-rare earth alloy. Metall Mater Trans A Phys Metall Mater Sci 2010;41:73-84. https://doi.org/10.1007/s11661-009-0080-2.
- [18] Luo XC, Kang LM, Liu HL, Li ZJ, Liu YF, Zhang DT, et al. Enhancing mechanical properties of AZ61 magnesium alloy via friction stir processing: Effect of processing parameters. Materials Science and Engineering A 2020;797. https://doi.org/10.1016/j.msea.2020.139945.
- [19] Karthikeyan L, Senthilkumar VS, Balasubramanian V, Arul S. Analysis of first mode metal transfer in A413 cast aluminum alloy during friction stir processing. Mater Lett 2010;64:301-4. https://doi.org/10.1016/j.matlet.2009.10.068.
- [20] Sun N, Apelian D. Friction Stir Processing of Aluminum Cast Alloys for High Performance Applications. JOM 2011;63:44-50. https://doi.org/10.1007/s11837-011-0190-3.
- [21] Nakata K, Kim YG, Fujii H, Tsumura T, Komazaki T. Improvement of mechanical properties of aluminum die casting alloy by multi-pass friction stir processing. Materials Science and Engineering A 2006;437:274-80. https://doi.org/10.1016/j.msea.2006.07.150.
- [22] Samanta A, Seffens RJ, Das H, Guzman A, Roosendaal TJ, Garcia D, et al. Microstructure-refinement-driven enhanced tensile properties of high-pressure die-cast A380 alloy through friction stir processing. J Manuf Process 2022;78:352–62. https://doi.org/10.1016/j.jmapro.2022.04.027.
- [23] Santella ML, Engstrom T, Storjohann D, Pan TY. Effects of friction stir processing on mechanical properties of the cast aluminum alloys A319 and A356. Scr Mater 2005;53:201-6. https://doi.org/10.1016/j.scriptamat.2005.03.040.
- [24] Rao AG, Deshmukh VP, Prabhu N, Kashyap BP. Ductilizing of a brittle as-cast hypereutectic Al-Si alloy by friction stir processing. Mater Lett 2015;159:417-9. https://doi.org/10.1016/j.matlet.2015.07.006.
- [25] Ma ZY, Pilchak AL, Juhas MC, Williams JC. Microstructural refinement and property enhancement of cast light alloys via friction stir processing. Scr Mater 2008;58:361-6. https://doi.org/10.1016/j.scriptamat.2007.09.062.
- [26] Nelaturu P, Jana S, Mishra RS, Grant G, Carlson BE. Influence of friction stir processing on the room temperature fatigue cracking mechanisms of A356 aluminum alloy. Materials Science and Engineering A 2018;716:165-78. https://doi.org/10.1016/j.msea.2018.01.044.
- [27] Tajiri A, Uematsu Y, Kakiuchi T, Tozaki Y, Suzuki Y, Afrinaldi A. Effect of friction stir processing conditions on fatigue behavior and texture development in A356-T6 cast aluminum alloy. Int J Fatigue 2015;80:192–202. https://doi.org/10.1016/j.ijfatigue.2015.06.001.
- [28] Abubaker HM, Merah N, Al-Badour F, Sorour AA, Ul-Hamid A, Kumar AM, et al. Influence of Friction Stir Processing on Wear, Corrosion, and Fracture Toughness Behavior of 2507 Super Duplex Stainless Steel. J Mater Eng Perform 2021;30:89–102. https://doi.org/10.1007/s11665-020-05325-4.
- [29] Samanta A, Das H, Grant GJ, Jana S. Effect of tool design and pass strategy on defect elimination and uniform, enhanced tensile properties of friction stir processed high-pressure die-cast A380 alloy. Materials Science and Engineering: A 2022;861:144388. https://doi.org/10.1016/j.msea.2022.144388.
- [30] ASTM standard, Designation: B871-01, Standard Test Method for Tear Testing of Aluminum Alloy Products (Current edition approved April 1, 2007. Published May 2007. Originally approved in 1996. Last previous edition approved in 2001 as B 871-01). 2007.
- [31] Yang KV, Cáceres CH, Nagasekhar A v., Easton MA. The skin effect and the yielding behavior of cold chamber high pressure die cast Mg-Al alloys. Materials Science and Engineering A 2012;542:49-55. https://doi.org/10.1016/j.msea.2012.02.029.
- [32] Xia Y, Zheng J, Chen J, Zhang Y, Shi R, Zhou H, et al. The Ductility Variation of High-Pressure Die-Cast AE44 Alloy: The Role of Inhomogeneous Microstructure. Metall Mater Trans A Phys Metall Mater Sci 2021;52:2274-86. https://doi.org/10.1007/s11661-021-06220-w.
- [33] Bauchau OA, Craig JI. Euler-Bernoulli beam theory, 2009, p. 173-221. https://doi.org/10.1007/978-90-481-2516-6_5.
9. Copyright:
- This material is a paper by "Avik Samanta, Hrishikesh Das, Glenn J. Grant, Saumyadeep Jana". Based on "Friction Stir Processing: A Thermomechanical Processing Tool for High Pressure Die Cast Al-Alloys for Vehicle Light-weighting".
- Source of the paper: https://doi.org/10.1016/j.mfglett.2024.504512
This material is summarized based on the above paper, and unauthorized use for commercial purposes is prohibited.
Copyright © 2025 CASTMAN. All rights reserved.