This article introduces the paper "A water-soluble core material for manufacturing hollow composite sections presented in Composite Structures".
1. Overview:
- Title: A water-soluble core material for manufacturing hollow composite sections
- Authors: Z. Xiao, L.T. Harper, A.R. Kennedy, N.A. Warrior
- Publication Year: 2017
- Publishing Journal/Academic Society: Composite Structures
- Keywords: Compression moulding, Water-soluble, Core, Carbon fibre composite

2. Research Background:
Social/Academic Context of the Research Topic
Core materials play a crucial role in composite structures by enhancing the second moment of area and improving bending stiffness. While foam materials are commonly used for sandwich panels, high-volume composite manufacturing methods like compression moulding or High-Pressure Resin Transfer Moulding (HP-RTM) can generate significant pressures (30-150 bar). These pressures can crush conventional closed-cell foams unless they possess very high densities, leading to increased weight. Removable core materials offer an alternative, frequently employed in manufacturing hollow metallic structures, such as sand cores in casting processes. However, existing removable cores often face challenges in high-pressure moulding scenarios, resin infiltration, efficient removal, cost-effectiveness, and environmental considerations.
Limitations of Existing Research
Current removable core materials present several limitations:
- Sand cores: Require mechanical removal, which can be challenging for intricate geometries or delicate components.
- Extractable polymers and low melting temperature metals: Have removal temperatures that may be incompatible with polymer matrices or lack sufficient stiffness during moulding.
- Caustic solutions for ceramic cores: Pose environmental hazards and can negatively impact the performance of the final product.
- Resin infiltration: Many current removable cores are susceptible to resin infiltration during high-pressure moulding, making core removal difficult.
Necessity of the Research
There is a clear need for a low-cost, easily removable core material that can:
- Withstand high pressures encountered during composite moulding.
- Be impermeable to low-viscosity resins.
- Be removed quickly and easily without causing damage to the composite structure.
This research addresses these needs by exploring a water-soluble sodium chloride (NaCl) core for producing hollow carbon fibre composites.
3. Research Purpose and Research Questions:
Research Purpose
This research investigates the potential of utilizing water-soluble sodium chloride (NaCl) cores, bonded with a water-soluble trehalose sugar, for manufacturing hollow carbon fibre composites using high-pressure moulding processes. The primary aim is to define a processing window for these NaCl cores to achieve:
- Suitable mechanical properties: For use in high-pressure moulding (100-150 bar).
- Efficient core removal: From the composite part after moulding.
Key Research Questions
- What is the compressive strength of the developed NaCl core material at both ambient and elevated temperatures relevant to composite moulding (e.g., 120 °C)?
- How do factors like binder content, processing pressure, temperature, and time affect the mechanical properties (compressive and flexural strength), dissolution rate, and density of the NaCl core?
- Can the NaCl core be effectively removed from a composite component after high-pressure moulding, and what is its dissolution rate?
- Is the NaCl core machinable for creating complex geometries and cost-effective for high-volume applications?
Research Hypotheses
The study hypothesizes that an optimized mixture of NaCl and trehalose binder can create a core material with:
- Sufficient compressive strength: To endure high-pressure moulding.
- Resin impermeability: To prevent resin infiltration.
- Easy water removal: For efficient core removal post-moulding.
It is also hypothesized that the mechanical properties and dissolution rate of the core can be adjusted by controlling binder content, processing pressure, temperature, and time.
4. Research Methodology
Research Design
The research employed an experimental approach using:
- Taguchi study: To optimize processing parameters.
- Full-factorial study: To evaluate the impact of in-service temperatures.
Data Collection Method
Various experimental methods were used to characterize the developed core material (NT Core) and benchmark materials:
- Mechanical Testing:
- Compressive strength: Measured using compression tests.
- Flexural strength: Measured using 3-point bending tests.
- Microscopy: Optical micrographs were used to examine core material cross-sections.
- Thermoanalytical Testing:
- Coefficient of Thermal Expansion (CTE): Measured using TMA.
- Phase transitions of trehalose binder: Analyzed using DSC.
- Dissolution Rate Measurements: Ultrasonic water bath and conductivity meter were used to measure dissolution rates.
- Machining Trials: Slots were machined using ball nose and flat end mills to assess machinability.
- Core Removal Trials: Water jet removal trials were conducted to compare removal rates.
Analysis Method
Data analysis methods included:
- Taguchi Method Analysis: To identify significant processing parameters.
- Statistical Analysis: To quantify parameter influence using mean effects and change ratios.
- Stress-Strain Curve Analysis: To understand material behavior and failure modes.
- DSC Curve Analysis: To characterize trehalose phase transitions.
- Dissolution Rate Calculation: Using a derived formula based on conductivity changes.
- Surface Roughness Measurement: To assess surface quality after machining.
- Volume Measurement: To quantify core volume removed during water jet trials.
Research Subjects and Scope
The research focused on a water-soluble core material (NT Core) composed of sodium chloride (NaCl) and trehalose binder. The scope included:
- Optimization: Core material composition and processing parameters.
- Evaluation: Mechanical properties at ambient and elevated temperatures.
- Assessment: Dissolution rate, density, CTE, and machinability.
- Comparison: Performance against benchmark materials (A Core and B Core).
- Demonstration: Core removal process in a hollow composite section.
5. Main Research Results:
Key Research Results
- High Compressive Strength: The NaCl core achieved a compressive strength of 57 MPa at ambient temperature and 20 MPa at 120 °C, outperforming commercial benchmarks.
- Processing Pressure Dominance: Processing pressure was the most significant factor influencing mechanical strength.
- Binder Content Influence: Binder content positively affected mechanical properties up to 8 wt%.
- Temperature and Time Effects: Processing temperature and time improved mechanical properties due to trehalose phase transformations, with 150 °C identified as optimal.
- Controllable Dissolution Rate: The specific dissolution rate (SDR) ranged from 0.14 to 1.23 kg/(min-m²), influenced by processing parameters.
- CTE Compatibility: The CTE of the NT Core was approximately 43 × 10⁻⁶/K, similar to sandwich panel cores.
- Machinability Demonstrated: NT Core showed good machinability, especially with ball-nose cutters.
- Successful Water Jet Removal: Water jet removal trials demonstrated effective core removal.
Statistical/Qualitative Analysis Results
- Processing Pressure Significance: Taguchi analysis confirmed processing pressure as the most influential factor for mechanical strength.
- Parameter Effects: Increased binder content, processing temperature, and time generally improved mechanical properties but reduced SDR.
- Trehalose Phase Transitions: DSC analysis confirmed trehalose phase transitions during processing, impacting core properties.
- Porosity Reduction: Microscopy revealed reduced porosity at higher processing temperatures, correlating with improved strength.
Data Interpretation
- Mechanical Interlocking: Increased processing pressure enhances mechanical interlocking of salt grains and reduces porosity, leading to higher strength.
- Trehalose Binder Mechanism: Trehalose binder acts as an adhesive, and its phase transformation at higher temperatures further strengthens the core by filling voids and creating a denser structure.
- Strength-Dissolution Trade-off: A trade-off exists between mechanical strength and dissolution rate, with denser cores being stronger but dissolving slower.
- NT Core Suitability: The NT Core demonstrates a good balance of mechanical properties and dissolution rate, making it suitable for high-pressure composite moulding.
Figure Name List
- Fig. 1. Cutting plan of test specimens from a circular disc, 150 mm diameter, 13 mm thick.
- Fig. 2. Schematics of two types of cutting slots, scanning direction and range of 50 mm (a); 6 locations where the inductive gauge places (b) on a round sample disc with 150 mm diameter.
- Fig. 3. Schematics of core dimensions (a), core wrapped with composites shell (b), and positions of water jet nozzle and wrapped core (c) in water removal trial.
- Fig. 4. Compressive (a) and flexural (b) stress/strain curves for NT Cores with different binder levels and processing temperatures (manufactured at 170 bar for 10 min).
- Fig. 5. DSC profile of trehalose from 20 °C to 230 °C (heating rate 5 °C/min).
- Fig. 6. DSC profiles of trehalose from two heating process in succession within 20 °C-150 °C.
- Fig. 7. Optical microscope images of cross sections of the NT Cores having 8 wt% binder content and processed under 170 bar at (a) 120 °C for 10 min (porosity:16%) and (b) 190 °C for 10 min (porosity: 10%).
- Fig. 8. Degree of dissolution for NT Core samples in the Taguchi study (legend shows: processing pressure-binder content-processing temperature-processing time).
- Fig. 9. Compressive strengths of NT Cores manufactured using different temperatures and times (different line colours) and tested at 20 °C (solid line) and 120 °C (dotted line).
- Fig. 10. Flexural strengths of NT Cores made at various temperatures and times (different line colours) and tested at 20 °C (solid line), 80 °C (dashed line) and 120 °C (dotted line).
- Fig. 11. Different colours of NT Cores made at various temperatures for different time.
- Fig. 12. The NT Core after end-milling with a Ø10 mm ball-nose cutter (a), a Ø10 mm flat cutter (b).
- Fig. 13. The change in volume of B Core and NT Core (8 wt % binder content, pressed at 150 °C and 170 bar for 30 min) during water jet trials.
- Fig. 14. An open-ended integrally-stiffened prepreg panel (a) NT Core in position and (b) after NT Core removal, showing blind hole.
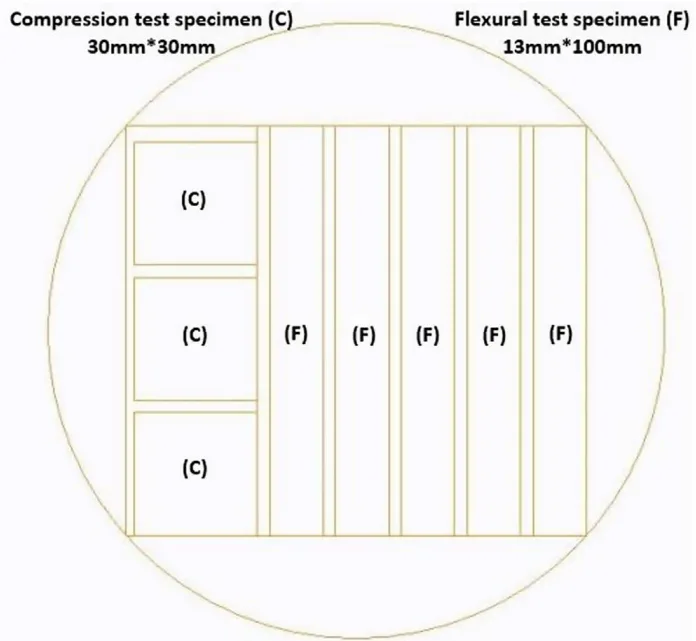
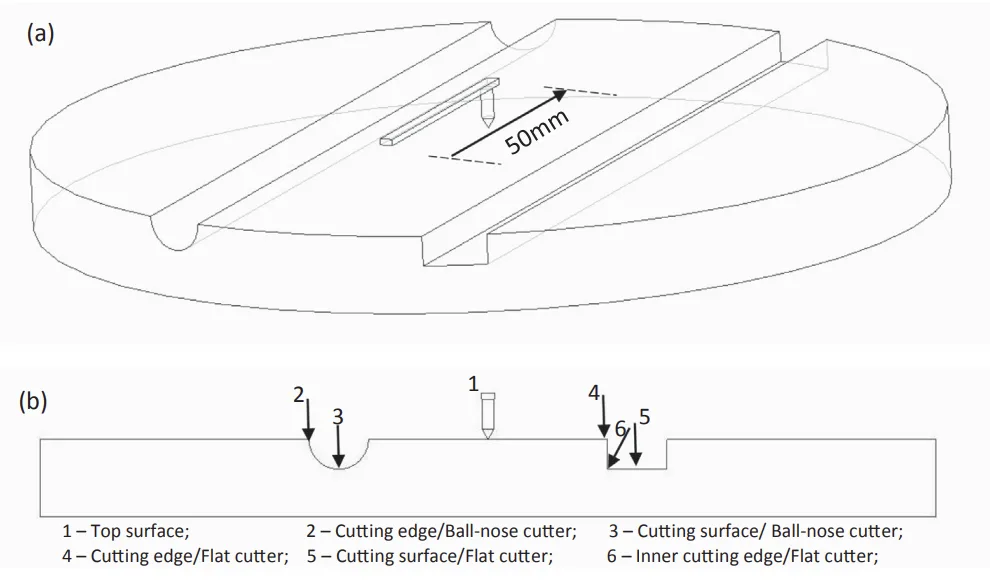
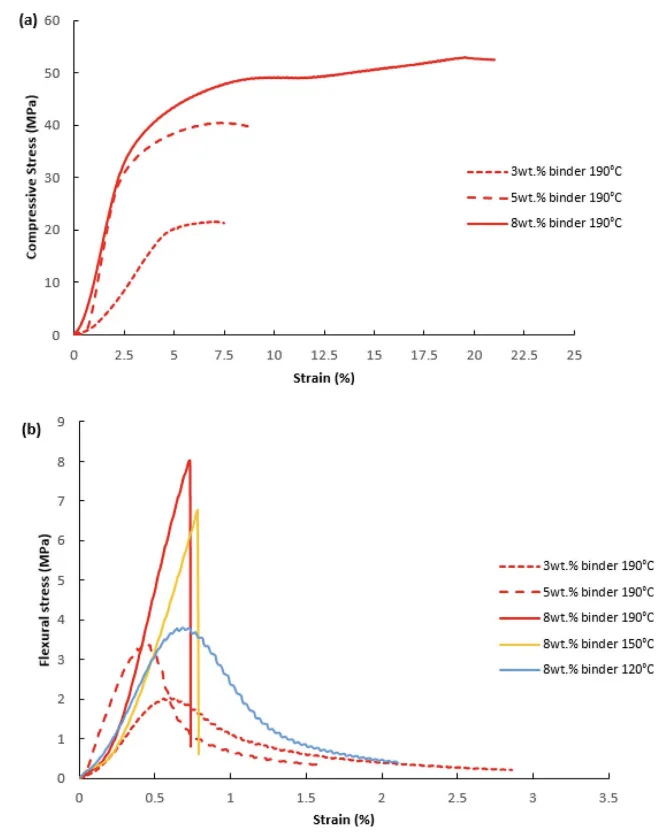
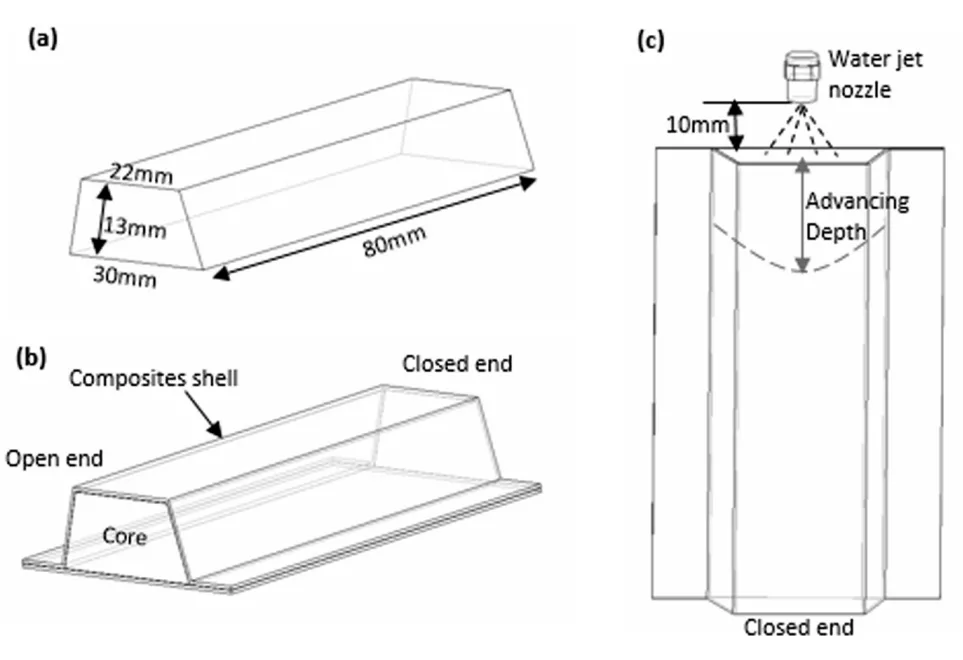
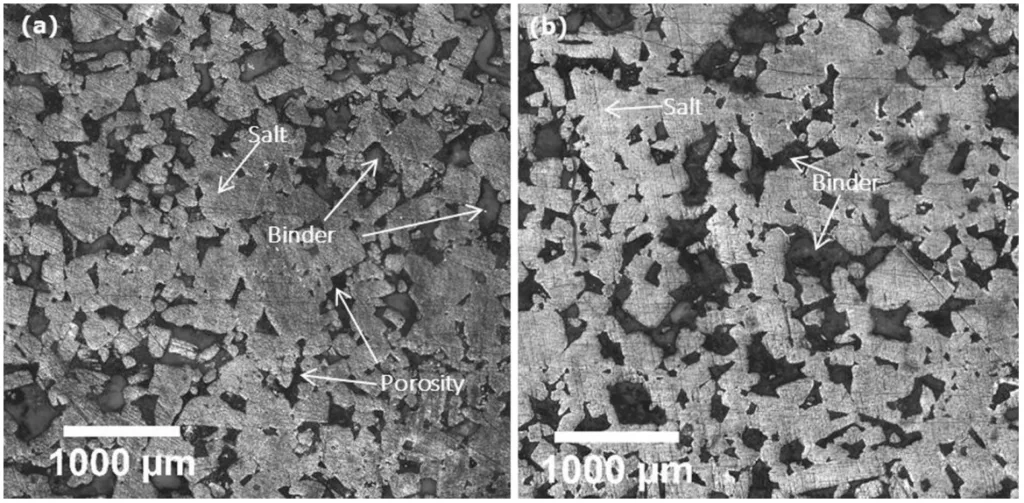
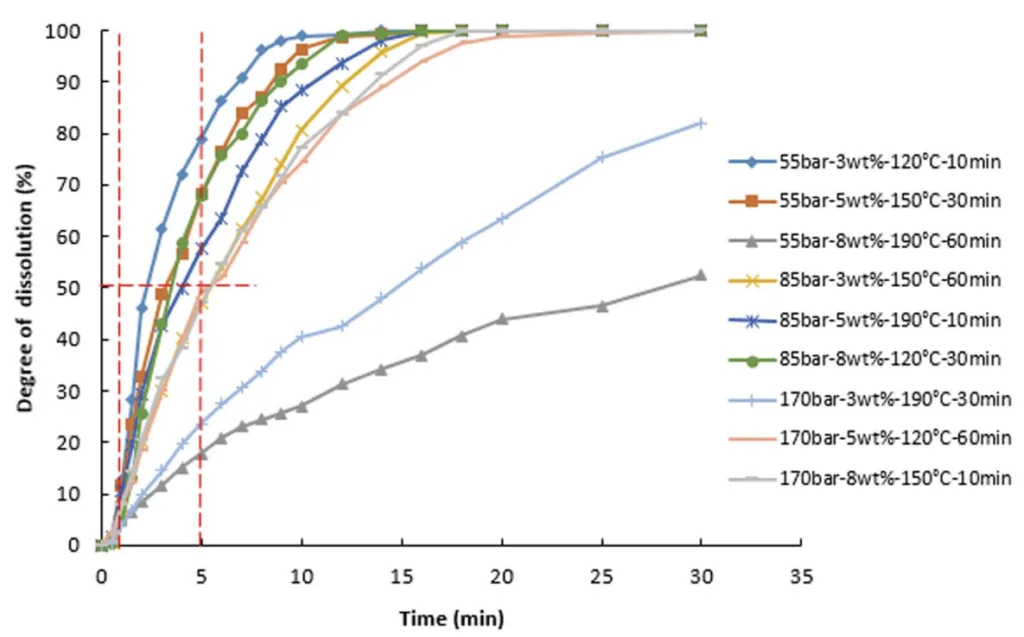
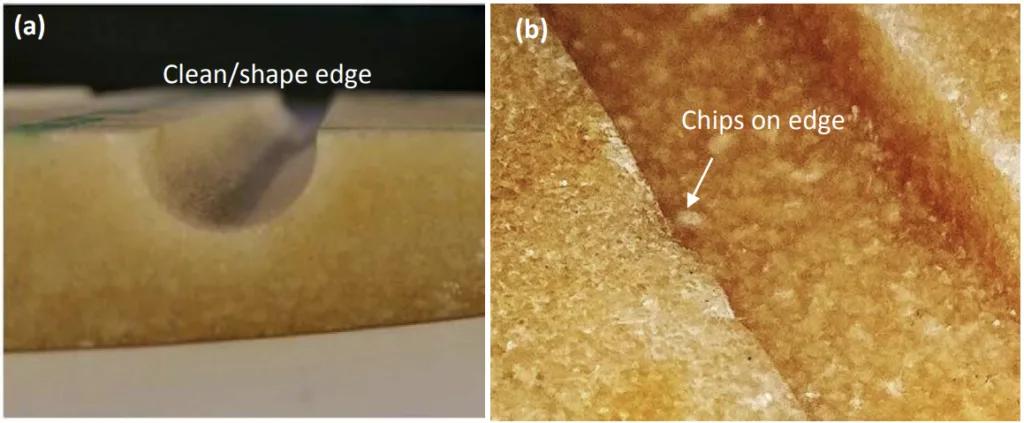
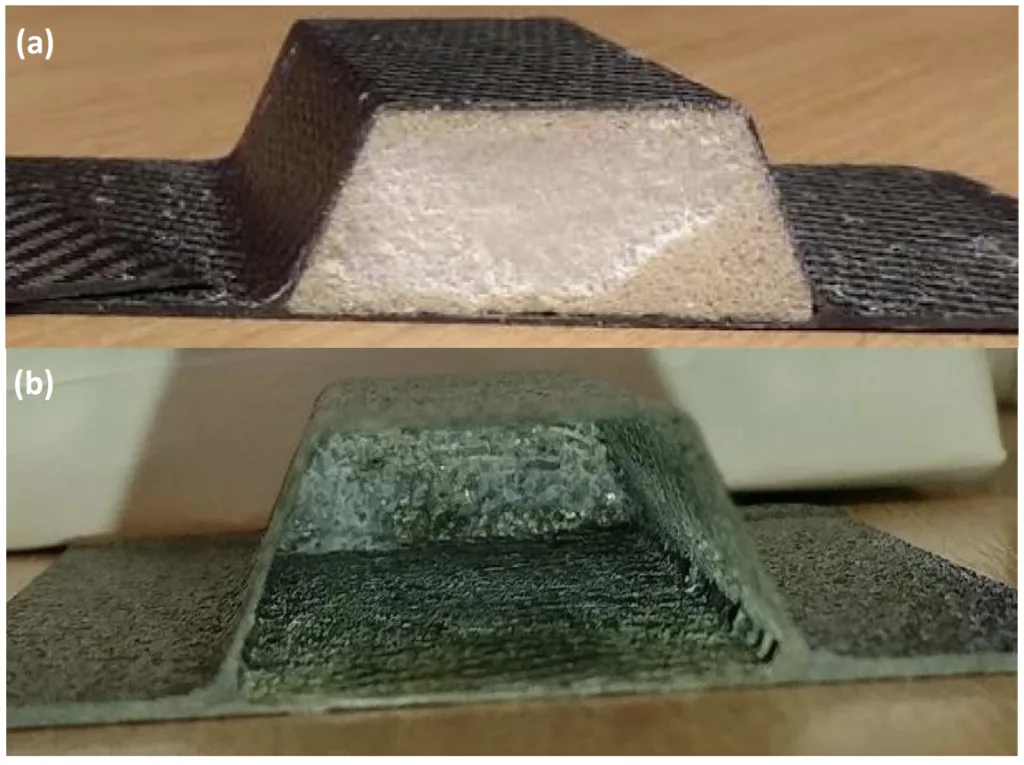
6. Conclusion and Discussion:
Summary of Main Results
The research successfully developed a water-soluble NaCl-based core (NT Core) using trehalose binder, suitable for high-pressure composite moulding. The NT Core demonstrated:
- High mechanical strength: Compressive strength of 57.3 MPa at 20 °C and 19.7 MPa at 120 °C; flexural strength of 10.2 MPa at 20 °C and 3.2 MPa at 120 °C.
- Optimized properties: Mechanical properties were optimized by adjusting processing parameters, particularly pressure and temperature.
- Controllable dissolution: The core exhibited a controllable dissolution rate and good machinability.
- Successful removal: Effective removal from composite parts using water jet was demonstrated.
Academic Significance of the Research
This research makes a significant academic contribution by:
- Introducing a viable, low-cost, water-soluble core material: For high-pressure moulding of composites.
- Providing detailed understanding: Of the relationship between processing parameters, mechanical properties, and dissolution behavior of NaCl-trehalose cores.
- Elucidating the role of trehalose binder: In enhancing core performance through phase transitions.
Practical Implications
The NT Core offers several practical advantages:
- Practical alternative: To existing removable core materials for manufacturing complex hollow composite structures.
- Water solubility: Enables easy and environmentally friendly removal.
- Machinability: Allows for creating complex geometries.
- High strength at elevated temperatures: Suitable for high-pressure RTM and compression moulding.
- Low cost: Economically attractive due to the use of NaCl and trehalose.
Limitations of the Research
- Mechanical property reduction at 120 °C: Needs consideration for high-temperature moulding applications.
- Potential resin infiltration: Suggests the need for a coating layer for certain resin systems or processing conditions.
- Slower water jet removal rate: Compared to the benchmark B Core, indicating room for optimization.
- Limited scope: Focused on a specific composition and processing window, requiring further exploration.
7. Future Follow-up Research:
Directions for Follow-up Research
- Investigate coating methods to prevent resin infiltration.
- Optimize water jet removal parameters to improve removal rate.
- Explore recyclability of dissolved NaCl and trehalose.
- Conduct further machining trials for complex geometries.
- Evaluate performance in actual composite component manufacturing.
- Investigate long-term stability and shelf life of the NT Core.
- Explore alternative binder materials and compositions.
Areas Requiring Further Exploration
- Effect of different resin systems and moulding cycles on core performance.
- Scale-up manufacturing for industrial applications.
- Cost-benefit analysis compared to other core materials.
- Environmental impact assessment of NT Core.
8. References:
- [1] Graf M, et al. High pressure resin transfer moulding-process advancements. In: 10th ACCE 2010.
- [2] Ritter K. RTM advances facilitate mass production in the automotive market. 2012 Available from: http://www.materialstoday.com/composite-applications/features/rtm-advances-facilitate-mass-production-in-the/.
- [3] Zajonz A, Stoger W. In-mold foamed cores for lightweight design. 2015 Available from: http://www.rohacell.com/sites/lists/PP-HP/Documents/KUint_2015_10%20In-Mold%20Foamed_.pdf.
- [4] Jelinek P, Adamkova E. Lost cores for high-pressure die casting. Arch Foundry Eng 2014;14(2/2014):101-4.
- [5] Michels H, Bunck M, Buhrig-Polaczek A. Suitability of lost cores in rheocasting process. Trans Nonferrous Met Soc China 2010;20:948-53.
- [6] LaFay V. Application of no-bake sodium silicate binder systems. Int J Metalcast 2012;6(3):19-26.
- [7] Jiang W, et al. Preparation and properties of a novel water soluble core material. J Mater Sci Technol 2010;26:270-5.
- [8] Sangeeta D, Niskayuna NY. Method of dissolving or leaching ceramic cores in airfoils. US: General Electric Company; 1998.
- [9] Amaro AM, et al. Effects of alkaline and acid solutions on glass/epoxy composites. Polym Degrad Stab 2013;98:853-62.
- [10] Pierri D. Lost core: new perspectives in die casting. 2012 Available from: http://doc.assofond.it/congr2012/tecn/2BUHLER.pdf.
- [11] Yaokawa J, et al. Strength of salt core composed of alkali carbonate and alkali chloride mixtures made by casting technique. Mater Trans 2007;48(5):1034-41.
- [12] Jelinek P, et al. Development of foundry cores based on inorganic salts. Mater Technol 2013;47:689-93.
- [13] Edward BF, Paul GA. Process for making soluble cores. U.S. Patents; 1974.
- [14] Pierri D. Lost core neue Perspektiven im Druckguss. 2013 Available from: www.fhnw.ch/technik/forschung-und-entwicklung/giesserei-zentrum/giessereitag/giessereitag-2013/lost-core-neue-perspektiven-im-druckguss-dario-pierri-buhler-ag.pdf.
- [15] Taylor LS, York P. Characterization of the phase transitions of trehalose dihydrate on heating and subsequent dehydration. J Pharm Sci 1998;87(3):347-55.
- [16] Sussich F, et al. Polymorphic amorphous and crystalline forms of trehalose. J Am Chem Soc 1998;120:7893-9.
- [17] Furuki T, Kishi A, Sakurai M. De- and rehydration behavior of a, a-trehalose dihydrate under humidity-controlled atmospheres. Carbohydr Res 2005;340:429-38.
- [18] German RM. Powder metallurgy science. 105 College Rd. E, Princeton, NJ 08540, USA: Metal Powder Industries Federation; 1984. p. 279.
- [19] Rao ASM, et al. Thermophysical properties of NaCl, NaBr and NaF by y-ray attenuation technique. J Mod Phys 2013;04(02):208-14.
- [20] ROHACELL for the Automotive Industry. [cited 2016; Available from: http://www.rohacell.com/sites/lists/PP-HP/Documents/ROHACELL-Automotive-EN.pdf.
- [21] Newman S, Meyer FJ. Mica composites of improved strength. Polym Compos 1980;1(1):37-43.
- [22] Gunay M, et al. Investigation of the effect of rake angle on main cutting force. Int J Mach Tools Manuf 2004;44:953-9.
9. Copyright:
- This material is Z. Xiao, L.T. Harper, A.R. Kennedy, N.A. Warrior's paper: Based on A water-soluble core material for manufacturing hollow composite sections.
- Paper Source: http://dx.doi.org/10.1016/j.compstruct.2017.09.058
This material was summarized based on the above paper, and unauthorized use for commercial purposes is prohibited.
Copyright © 2025 CASTMAN. All rights reserved.