Kohlstädt, Sebastian
KTH, School of Industrial Engineering and Management (ITM), Materials Science and Engineering.
Vynnycky, Michael
KTH, School of Industrial Engineering and Management (ITM), Materials Science and Engineering.ORCID iD: 0000-0002-8318-1251
Neubauer, Alexander
Volkswagen AG, Div Components Mfg, Business Unit Casting, Dr Rudolf Leiding Pl 1, D-34225 Baunatal, Germany..
Gebauer-Teichmann, Andreas
Volkswagen AG, Div Components Mfg, Business Unit Casting, Dr Rudolf Leiding Pl 1, D-34225 Baunatal, Germany..
Abstract
In this work, the implementation of three turbulence models inside the open source C++ computational fluid dynamics (CFD) library OpenFOAM were tested in 2D and 3D to determine the viability of salt cores in high pressure die casting. A finite-volume and volume of fluid approach was used to model the two-phase flow of molten metal and air, with the latter being treated as compressible. Encouragingly, it is found that, although the choice of turbulence model seems to affect the dispersion of the two-phase interface, the force acting at the surface of the salt core depends only very weakly on the turbulence model used. The results were also compared against those obtained using the commercially available and widely-used casting software MAGMA(5).
Korea Abstract
이 작업에서 오픈 소스 C++ CFD(Computational Fluid Dynamics) 라이브러리 OpenFOAM 내부의 세 난류 모델 구현을 2D 및 3D로 테스트하여 고압 다이 캐스팅에서 염심의 생존 가능성을 결정했습니다. 유한 체적 및 유체 체적 접근법은 용융 금속 및 공기의 2상 유동을 모델링하는 데 사용되었으며 후자는 압축성으로 처리됩니다. 고무적으로, 난류 모델의 선택이 2상 계면의 분산에 영향을 미치는 것처럼 보이지만 염심 표면에 작용하는 힘은 사용된 난류 모델에 매우 약하게만 의존한다는 것이 발견되었습니다. 결과는 또한 상업적으로 이용 가능하고 널리 사용되는 주조 소프트웨어 MAGMA(5)를 사용하여 얻은 결과와 비교되었습니다.
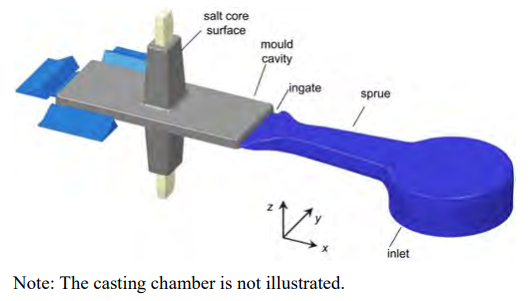
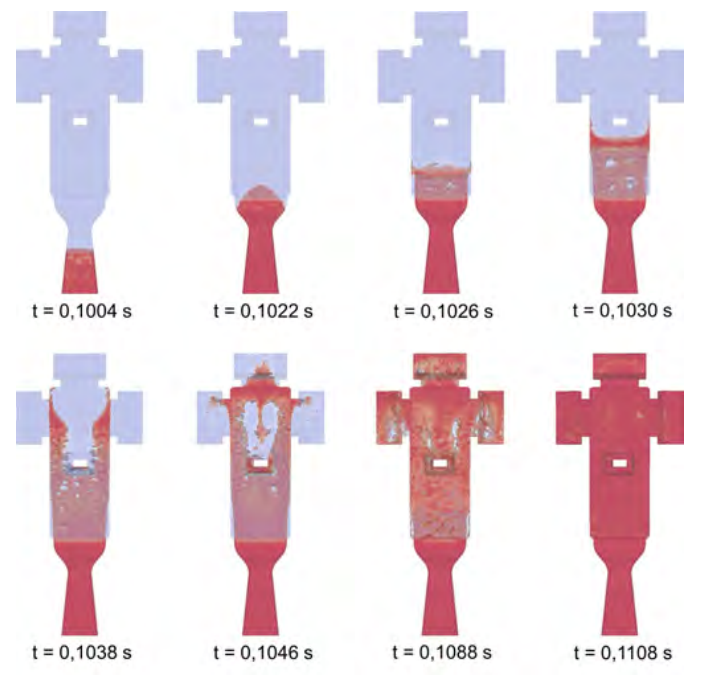
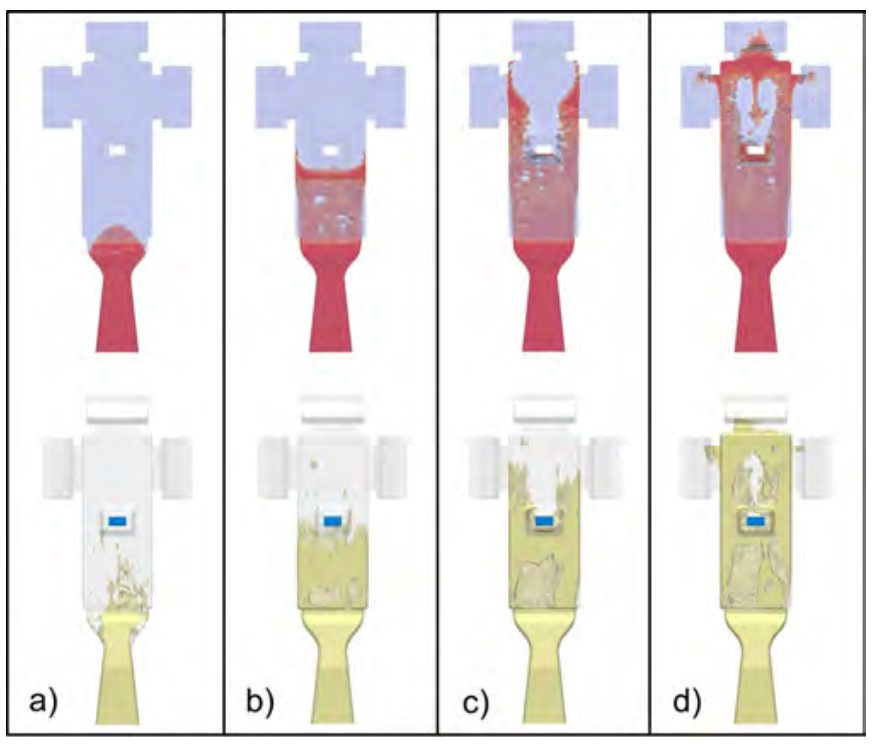
References
Beitz, W., Küttner, K.H. and Davies, B.J. (2013) DUBBEL –Handbook of Mechanical Engineering, Springer-VerlagLondon.
Brackbill, J.U., Kothe, D.B. and Zemach, C. (1992) ‘A continuum method for modeling surface tension’, Journal of Computational Physics, Vol. 100, No. 2, pp.335–354.
Cleary, P.W., Ha, J., Prakash, M. and Nguyen, T. (2006) ‘3D SPH flow predictions and validation for high pressure die casting of automotive components’, Applied Mathematical Modelling, Vol. 30, No. 11, pp.1406–1427.
Courant, R., Friedrichs, K. and Lewy, H. (1967) ‘On the partial difference equations of mathematical physics’, IBM Journal of Research and Development, Vol. 11, No. 2, pp.215–234.
Ferrer, P.J.M., Causon, D.M., Qian, L., Mingham, C.G. and Ma, Z.H. (2016) ‘A multi-region coupling scheme for compressible and incompressible flow solvers for two-phase
flow in a numerical wave tank’, Computers and Fluids, Vol. 125, pp.116–129.
Fuchs, B., Eibisch, H. and Körner, C. (2013) ‘Core viability simulation for salt core technology in high pressure die casting’, International Journal of Metalcasting, Vol. 7, No. 3,
pp.39–45.
Fuchs, B. and Körner, C. (2014) ‘Mesh resolution consideration for the viability prediction of lost salt cores in the high pressure die casting process’, Progress in Computational Fluid Dynamics, Vol. 14, No. 1, pp.24–30.
Ha, J., Cleary, P.W., Alguine, V. and Nguyen, T. (1999) ‘Simulation of die filling in gravity die casting using SPH and MAGMASoft’, Proceedings of the 2nd International
Conference on CFD in Minerals and Process Industries, Melbourne, Australia, pp.423–428.
Ha, J., Cleary, P.W., Prakash, M., Alguine, V., Nguyen, T. and Scott, C. (2003) ‘SPH, MAGMASoft and water analogue modellings of die filling of a servo piston’, Proceedings of the 2nd International Conference on CFD in Minerals and Process Industries, Melbourne, Australia, pp.587–592.
Hirt, C.W. and Nichols, B.D. (1981) ‘Volume of fluid (VOF) method for the dynamics of free boundaries’, Journal of Computational Physics, Vol. 39, No. 1, pp.201–225.
Jasak, H., Jemcov, A. and Tuković, ˇZ. (2007) ‘OpenFOAM: a C++ Library for Complex Physics Simulations’, Paper presented at the International Workshop on Coupled Methods
in Numerical Dynamics IUC, Dubrovnik, Croatia, 19–21 September, 20pp.
Jelínek, P. and Adámková, E. (2014) ‘Lost cores for high-pressure die casting’, Archives of Foundry Engineering, Vol. 14, No. 2, pp.101–104.
Koch, M., Lechner, C., Reuter, F., Köhler, K., Mettin, R. and Lauterborn, W. (2016) ‘Numerical modeling of laser generated cavitation bubbles with the finite volume and
volume of fluid method, using OpenFOAM’, Computers and Fluids, Vol. 126, pp.71–90.
Launder, B.E. and Spalding, D.B. (1974) ‘The numerical computation of turbulent flows’, Computer Methods in Applied Mechanics and Engineering, Vol. 3, pp.269–289.
Mayon, R., Sabeur, Z., Tan, M-Y. and Djidjeli, K. (2016) ‘Free surface flow and wave impact at complex solid structures’, Paper presented at the 12th International Conference on
Hydrodynamics, Egmond aan Zee, NL, 18–23 September, Paper ID: 154, 10pp. Menter, F.R. (1994) ‘2-equation eddy-viscosity turbulence models for engineering applications’, AIAA Journal, Vol. 32, No. 8, pp.1598–1605.
Menter, F.R., Kuntz, M. and Langtry, R. (2003) ‘Ten years of industrial experience with the SST turbulence model’, Turbulence, Heat and Mass Transfer, Vol. 4, pp.625–632.
Nogowizin, B. (2010) Theorie und Praxis des Druckgusses, Fachverlag Schiele & Schön.
Robertson, E., Choudhury, V., Bhushan, S. and Walters, D.K. (2015) ‘Validation of OpenFOAM numerical methods and turbulence models for incompressible bluff body flows’,
Comp. Fluids, Vol. 123, pp.122–145.
Spalart, P.R. and Allmaras, S.R. (1994) ‘A one-equation turbulence model for aerodynamic flows’, Recherche Aerospatiale, No. 1, pp.5–21.
Versteeg, H. and Malalasekera, W. (2007) An Introduction to Computational Fluid Dynamics: The Finite Volume Method, 2nd ed., Pearson.
Weller, H.G., Tabor, G., Jasak, H. and Fureby, C. (1998) ‘A tensorial approach to computational continuum mechanics using object-oriented techniques’, Computers in Physics, Vol. 12, No. 12, pp.620–631.
White, F.M. (2011) Fluid Mechanics, 7th ed., McGraw-Hill, New York.
Wilcox, D.C. (1988) ‘Reassessment of the scale determining equation for advanced turbulence models’, AIAA Journal, Vol. 26, No. 11, pp.1299–1310.
Yakhot, V., Orszag, S.A., Thangam, S., Gatski, T.B. and Speziale, C.G. (1992) ‘Development of turbulence models for shear flows by a double expansion technique’, Physics of Fluids A: Fluid Dynamics, Vol. 4, pp.1510–1520.
Yamada, Y. (2013) EP Patent No. 20090742774 (July 10, 2013)
Yaokawa, J., Miura, D., Anzai, K., Yamada, Y. and Yoshii, H. (2006) ‘Mechanical properties of salt core comprised of alkali carbonate and alkali chloride’, Journal of Japan Foundry Engineering Society, Vol. 78, pp.1510–1520.