Suraj R. Marathe Assam Don Bosco University, Carmo E. Quadros Assam Don Bosco University
Date Written: February 13, 2021
Abstract
A numerical simulation approach is proposed to predict the optimal parameter setting during high pressure die casting. The contribution from the optimal parameters, the temperature, showed more influence on the casting quality than the other parameters. This study’s outcome was beneficial for finding the solution for casting defects that occurs due to incorrect setting of process parameters in die casting. Thus, a combination of numerical optimisation techniques and casting simulation serves as a tool to improve the casting product quality in die casting industries. This paper aims to analyse and optimise critical parameters like injection pressure, molten metal temperature, holding time, and plunger velocity, contributing to the defects. In this research paper, an effort has been made to give optimal pressure, temperature, holding time, and plunger velocity parameters using ProCAST simulation software that uses finite element analysis technology. Numerical analysis for optimising the parameters by varying the temperature of molten metal, injection pressure, holding time, and plunger velocity, concerning solidification time at hot spots, is an essential parameter for studying the defect analysis in the simulated model.
Keywords: High Pressure Die Casting (HPDC), Parameters, ProCAST, Simulation, Six Sigma Quality
Korea
고압 다이캐스팅 중 최적의 파라미터 설정을 예측하기 위해 수치 시뮬레이션 접근 방식이 제안됩니다. 최적 매개 변수 인 온도의 기여도는 다른 매개 변수보다 주조 품질에 더 많은 영향을 미쳤습니다.
이 연구의 결과는 다이캐스팅 공정 매개 변수의 잘못된 설정으로 인해 발생하는 주조 결함에 대한 솔루션을 찾는데 도움이 되었습니다. 따라서 수치 최적화 기술과 주조 시뮬레이션의 조합은 다이 캐스팅 산업에서 주조 제품 품질을 향상시키는 도구 역할을 합니다.
이 백서는 사출 압력, 용융 금속 온도, 유지 시간 및 플런저 속도와 같은 중요한 매개 변수를 분석하고 최적화 하여 결함에 기여하는 것을 목표로 합니다. 이 연구 논문에서는 유한 요소 분석 기술을 사용하는 ProCAST 시뮬레이션 소프트웨어를 사용하여 최적의 압력, 온도, 유지 시간 및 플런저 속도 매개 변수를 제공하기 위해 노력했습니다.
핫스팟에서의 응고 시간과 관련하여 용융 금속의 온도, 사출 압력, 유지 시간 및 플런저 속도를 변경하여 매개 변수를 최적화하기위한 수치 해석은 시뮬레이션 된 모델에서 결함 분석을 연구하는 데 필수적인 매개 변수입니다.
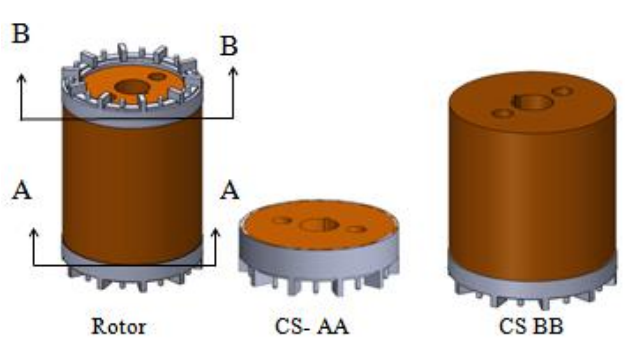
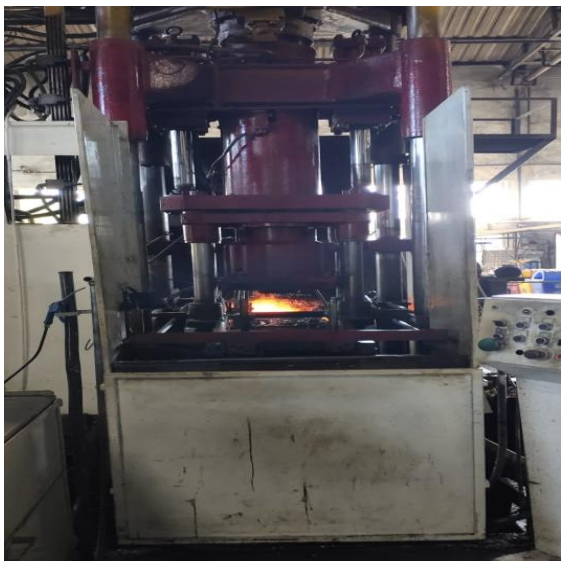
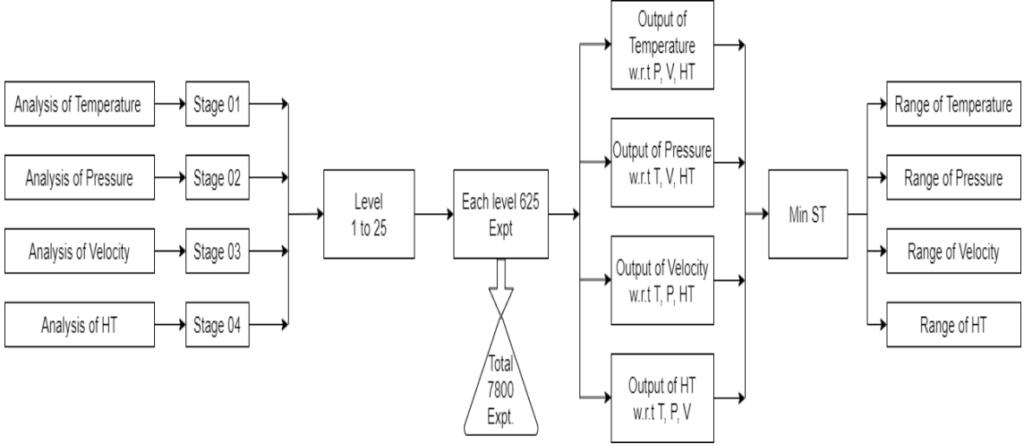
14 References
- K Domkin, J Hattel, J ThorborgPosted: 2009Crossref
- Modelling of high temperature and diffusion controlled die soldering in high aluminium pressure die castingJournal of Material Processing Technology, volume 209, issue 8, p. 4051 - 4061Crossref
- E Fiorese, D Richiedei, F BonolloImproving the quality of die castings through optimal plunger motion planning: analytical computation and experimental validationInternational Journal of Engineering and Management Research, volume 88, p. 1475 - 1484Posted: 2016Crossref
- Posted: 2021-02Crossref
- J Fu, K WangModelling and simulation of the die casting process for A356 semi-solid alloyProcedia Engineering, volume 81, p. 1565 - 1570Posted: 2014Crossref
- J Jorstad, D ApelianPressure assisted processes for high integrity aluminium castings -part 1International Journal of Metal Casting, p. 250 - 254Posted: 2009Crossref
- S Kumar, A Gupta, P ChandnaPosted: 2012Crossref
- Optimisation of process parameters of pressure die casting using taguchi methodologyWorld Academy of Science, Engineering and Technology, volume 6, p. 590 - 594Crossref
- L Lattanzi, A Fabrizi, A Fortini, M Merlin, G TimelliEffects of microstructure and casting defects on the fatigue behaviour of the high-pressure diecast AlSi9Cu3 (Fe) alloyProcedia Structural Integrity, volume 7, p. 505 - 512Posted: 2017Crossref
- C Mohanty, B JenaOptimisation of aluminium die casting process using artificial neural networkInternational Journal of Emerging Technology and Advanced Engineering, volume 4, issue 7, p. 146 - 149Posted: 2014
- G SyrcosDie casting process optimisation using taguchi methodsJournal of Material Processing Technology, volume 135, p. 68 - 74Posted: 2002Crossref
- L Wang, P Turnley, G SavageGas content in high pressure die castingsJournal of Materials Processing Technology, volume 211, p. 1510 - 1515Posted: 2011Crossref
- M Zhang, S Xing, L Xiao, P Bao, W Liu, Q XinDesign of process parameters for direct squeeze castingJournal of University of Science and Technology, volume 15, issue 3, p. 339 - 343Posted: 2008Crossref
- X Zhang, S Xiong, Q XuNumerical methods to improve the computational efficiency of solidification simulation for the investment casting processJournal of Materials Processing Technology, volume 173, p. 70 - 74Posted: 2006Crossref