This article introduces the paper "Optimization of the Surface Geometry in Structured Cold Rolling for Interlocking of Formed and Die-Cast Metal Components" published in Defect and Diffusion Forum in 2022.
1. Overview:
- Title: Optimization of the Surface Geometry in Structured Cold Rolling for Interlocking of Formed and Die-Cast Metal Components
- Authors: Aron Ringel and Johannes Lohmar
- Publication Year: 2022
- Publishing Journal/Academic Society: Defect and Diffusion Forum
- Keywords: interlocking, multi-material components, structured cold rolling, FE-modelling
2. Research Background:
- Social/Academic Context of the Research Topic:
Modern lightweight design is increasingly crucial, particularly in the automotive industry. Multi-material components are a key strategy for achieving weight reduction while maintaining performance and safety. Combining materials like steel and aluminium offers advantages, but joining them presents challenges due to potential chemical reactions, making traditional fusion welding unsuitable due to the formation of intermetallic phases with poor mechanical properties. Hybrid (or compound) casting, where liquid metal is joined to solid metal, emerges as a viable solution. For sheet metals, creating surface structures with undercuts through multi-pass rolling has proven effective for forming interlocking joints. However, the optimal surface structure for maximizing joint strength with these undercuts remains an open question. - Limitations of Existing Research:
While surface structures with undercuts created by multi-pass rolling are used for interlocking joints, the "best surface structure for these undercuts supporting the joint is still unknown." Existing research also notes that "inner notches can be prone to crack initiation and subsequently lead to component failure" under cyclic loading. - Necessity of the Research:
To address the limitations, this research is necessary to "understand the formation mechanisms of undercuts and inner notches to derive an optimum that maximizes undercuts while avoiding the formation of inner notches." This understanding is crucial for establishing "a sound understanding of the relation between the surface structure geometry and the achievable component strength."
3. Research Purpose and Research Questions:
- Research Purpose:
The primary research purpose is to "analyze the surface structure regarding channel depth, undercuts and inner notches as well as finally maximize the joint strength." A further aim is to "understand the formation mechanisms of undercuts and inner notches to derive an optimum that maximizes undercuts while avoiding the formation of inner notches." - Key Research Questions:
The research seeks to answer the question of optimizing surface geometry in structured cold rolling. Specifically, it aims to determine:- What surface geometry maximizes undercut width while preventing the formation of inner notches?
- What is the process optimum for creating surface structures with high undercut width but without inner notches?
- Research Hypotheses:
The research operates under the hypothesis that "the joint strength is decisively determined by the undercut geometry." It is also based on the observation that "the undercuts formed by material displacement increase with the height reduction in the flat rolling pass," but "after a certain amount of material displacement, the channel side edge starts to fold over the channel bottom and forms an inner notch."
4. Research Methodology:
- Research Design:
The study employs a "combined experimental and numerical study." This approach integrates "experimental and numerical study was laid out" to analyze the surface structure. - Data Collection Method:
Experimental data was collected using a "roll forming machine P3.160 by company Dreistern GmbH & Co. KG." Steel samples with different surface structures were prepared by "water jet for cross section preparation." The inner notch was made visible using "3% Nital etching for 30 s." The resulting structures were measured using a "Keyence VHX-1000D digital microscope." - Analysis Method:
Numerical analysis was performed using "2D explicit FE-model" simulations with the software Abaqus. The "evaluation of the results regarding undercut width and inner notch length was automated via a python script." Experimental results were analyzed by measuring the surface structures and correlating them with the process parameters. - Research Subjects and Scope:
The research focused on "2.0 mm thick steel sheet of type DC04." The surface was "structured with up to 0.5 mm deep channels and then flattened with different height reductions." Experiments used a sheet width of 160 mm and length of 245 mm. Simulations were simplified to a 2D plane strain model "covering height and width of half a channel and rib."
5. Main Research Results:
- Key Research Results:
The study yielded both simulation and experimental results regarding the optimization of surface geometry.- Simulation Results: "The results from the 2D explicit FE-model suggest that a process optimum for those surface structures with high undercut width but without inner notch exists at 14% height reduction."
- Experimental Results: "However, in the experiments inner notches started to form at approx. 8% height reduction with approx. 20 µm wide undercuts for the given experimental setup. In contrast, maximum undercuts of approx. 50 µm form at 26% height reduction, but also cause inner notches with approx. 60 µm length."
- "Notch free undercuts are only approx. 25 µm wide" in experiments.
- Statistical/Qualitative Analysis Results:
- Figure 3: "Example of 2D plane strain simulation with ɛh,1 = 12.08% and ɛh,2 = 28.18%" shows the material flow and stress distribution during the process, illustrating the formation of the channel and potential inner notch. It shows dimensions of "0.498 mm" channel depth, "0.142 mm" undercut width, and "0.358 mm" inner notch length for the given strain values.
- Figure 4: "Cross section of channel with undercut and inner notch, (ɛh,₁ = 18% and ɛh,2 = 33%)" presents a microscopic image of an experimental sample, visualizing the "undercut width" and "inner notch length." The scale bar indicates "50.00 μm."
- Figure 5: "Geometric parameters of simulation and experiment" graphically compares the simulated and experimental results for "channel rolling (1st)" and "flattening (2nd)." It plots "channel depth [mm]" and "undercut width [mm]" and "inner notch length [mm]" against "height reduction [%], Eq. (1)" and "height reduction [%], Eq. (2)" respectively. It shows that simulations predict undercut starting at approximately 7% height reduction and reaching a maximum of approximately 60 µm at 14% height reduction. Experiments show maximum undercut width of approximately 50 µm at approximately 26% height reduction, with inner notches appearing at approximately 8% height reduction.
- Data Interpretation:
The simulation results indicate an optimal height reduction of 14% for maximizing undercut width without inner notches. However, experimental results show that inner notches appear earlier and that maximum undercut width is achieved at a higher height reduction (26%) but accompanied by inner notches. "For the channel rolling pass, the plane strain simulation is overestimating the channel depth compared to the experimental results." This discrepancy is attributed to the "longitudinal material flow during channel rolling is not suppressed entirely by the undeformed side of the sample" in experiments, which is constrained in the 2D plane strain simulation. "Due to the plane strain restriction, the slope of the simulation line is approx. 0.04 mm per percent of height reduction. Because of the material flow in longitudinal direction, the experimental slope is only 65% as big." - Figure Name List:
- Fig. 1: Process scheme (as proposed by Senge et al.), [12]
- Fig. 2: Assembly of 2D simulation
- Fig. 3: Example of 2D plane strain simulation with ɛh,1 = 12.08% and ɛh,2 = 28.18%
- Fig. 4: Cross section of channel with undercut and inner notch, (ɛh,₁ = 18% and ɛh,2 = 33%)
- Fig. 5: Geometric parameters of simulation and experiment
- Fig. 6: Flow curve of DC04
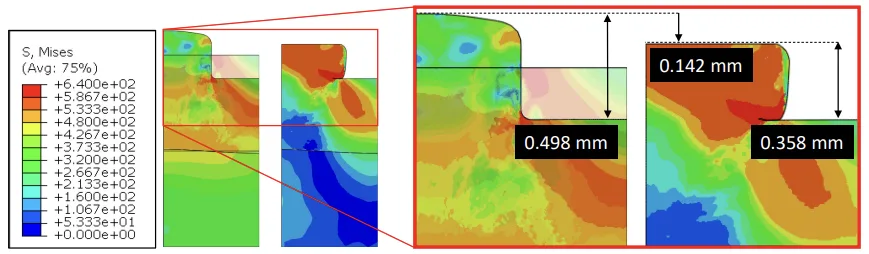
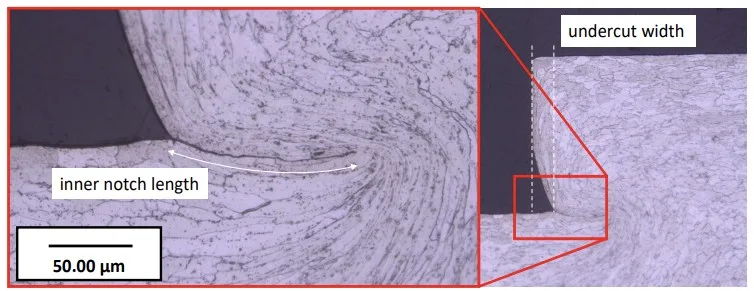
6. Conclusion and Discussion:
- Summary of Main Results:
"In this work, simulations and experiments have been conducted to maximize the surface structure geometry of a DC04 sheet for notch free undercuts." Simulations suggest "an optimum point at approx. 14% height reduction for 2 mm DC04 and the selected roller geometry" for notch-free undercuts. Experiments achieved "undercuts with up to 50 µm max. width" at 26% height reduction, but these were "with inner notches of approx. 60 µm." "Notch free undercuts are only approx. 25 µm wide" in the experimental setup and occurred at lower height reductions where inner notches were avoided. "This behaviour is currently not reproduced by the simulations." - Academic Significance of the Research:
The research contributes to the understanding of the "formation mechanisms of undercuts and inner notches" in structured cold rolling. It highlights the complexities of material flow and the challenges in accurately predicting surface geometry in these processes using 2D simulations. - Practical Implications:
The findings provide valuable insights for optimizing the surface geometry of sheet metals for "interlocking joints in multi-material components," particularly in the automotive industry. The research suggests that achieving maximum undercut width without inner notches requires careful control of height reduction in the cold rolling process. - Limitations of the Research:
"One way to improve accuracy and maintain efficacy is to add kinetic boundary conditions to the 2D plane strain model." The "2D plane strain model has limitations" in fully capturing the material flow, especially the longitudinal flow observed in experiments. "Simulations do not fully reproduce experimental results regarding inner notch formation."
7. Future Follow-up Research:
- Directions for Follow-up Research:
The authors suggest several directions for future research to improve the accuracy and applicability of the findings:- "One way to improve accuracy and maintain efficacy is to add kinetic boundary conditions to the 2D plane strain model."
- "Alternatively, a computationally expensive 3D model, including the undeformed sheet at the sides, would precisely describe the kinematic and stress state and allow for the investigation of friction effects."
- "For high accuracy, also the used flow curve extrapolation seems to play a major role."
- "Additionally, the influence of the roller geometry in the channel rolling pass should be investigated, as potentially the formation of inner notches in the flattening pass can be delayed, when larger fillet radii at the rib edge of the structured roller are used."
- "Besides, the undercut width might be increasing with wider ribs in the channel rolling pass."
- Areas Requiring Further Exploration:
Further exploration is needed in:- "Influence of roller geometry"
- "Friction effects"
- "3D modeling for better accuracy"
- "In future work the influence of this structure geometry on joint strength is to be tested using the rolled/die-cast composite component, to assess the simulation accuracy required from a practical standpoint."
8. References:
- [1] H.E. Friedrich, Leichtbau in der Fahrzeugtechnik, Springer Fachmedien, Wiesbaden, 2017.
- [2] B. Klein, T. Gänsicke, Leichtbau-Konstruktion, Springer Fachmedien, Wiesbaden, 2019.
- [3] W. Cai, G. Daehn, A. Vivek, J. Li, H. Khan, R.S. Mishra, M. Komarasamy, A State-of-the-Art Review on Solid-State Metal Joining, J. Manuf. Sci. Eng. 141 (3) (2019).
- [4] M. Rübner, M. Günzl, C. Körner, R.F. Singer, Aluminium-aluminium compound fabrication by high pressure die casting, Mater. Sci. Eng. A 528 (22-23), (2011) 7024–7029.
- [5] A. Bührig-Polaczek, T. Röth, E. Baumeister, N. Nowack, T. Süßmann, Hybride Leichtbaustrukturen in Stahlblech-Leichtmetall Verbundguss, FH Aachen (2006).
- [6] S. Senge, J. Brachmann, G. Hirt, A. Bührig-Polaczek, Evaluation of interlocking bond strength between structured 1.0338 steel sheets and high pressure die cast AlMg5Si2, in ESAFORM, (2018) p. 40019.
- [7] X. Fang, Evaluation of Coating Systems for Steel Aluminum Hybrid Casting; Mater. Sci. Eng. A 7 (2) (2017).
- [8] S. Ucsnik, R. Gradinger, A. Becirovic, A. Waldhör, Enhanced Performance of Steel-Aluminium Cast Nodes through Cold Metal, Transfer. Mater. Sci. Forum 765, (2013) 736–740.
- [9] N. Pasligh, Hybride formschlüssige Strukturverbindungen in Leichtbaustrukturen aus Stahlblech und Aluminiumdruckguss, Dissertation, Gießerei-Institut, Aachen (2011).
- [10]D. Joop, S. Heupel, C. Schnatterer, D. Zander, A. Bührig-Polaczek, Evaluation of Corrosion Mechanisms of Hybrid Light Metal Structures by High Pressure Die Casting, Mater. Sci. Forum 825-826, (2015) 441-448.
- [11]J. Jakumeit, H. Behnken, R. Laqua, J. Eiken, J. Brachmann, Multi-scale simulation of hybrid light metal structures produced by high pressure die casting; IOP Conf. Ser.: Mater. Sci. Eng. 861, (2020) p. 12035.
- [12] S. Senge, J. Brachmann, G. Hirt, A. Bührig-Polaczek, Interlocking Multi-Material Components made of Structured Steel Sheets and High-Pressure Die Cast Aluminium, in ESAFORM, (2017) p. 190007.
- [13]S. Senge, Walztechnische Herstellung von Oberflächenstrukturen für formschlüssige Verbindungen, Dissertation, Verlagshaus Mainz GmbH, Aachen (2020).
- [14] Y. Iino, Local Fatigue Damage Accumulation around Notch Attending Crack Initiation, Metall. Mater. Trans. A (26A), (1995) p. 1419.
- [15] A. Tieu, Y. Liu, Friction variation in the cold-rolling process, Tribol. Int. 37 (2), (2004) p. 177.
- [16] T. Clausmeyer, A. Schowtjak, S. Wang, R. Gitschel, O. Hering, P. Pavliuchenko, J. Lohmar, R. Ostwald, G. Hirt, A.E. Tekkaya, Prediction of Ductile Damage in the Process Chain of Caliber Rolling and Forward Rod Extrusion, Procedia Manuf. 47 (1), (2020) p. 649–655.
9. Copyright:
- This material is Aron Ringel and Johannes Lohmar's paper: Based on Optimization of the Surface Geometry in Structured Cold Rolling for Interlocking of Formed and Die-Cast Metal Components.
- Paper Source: doi:10.4028/p-z54p05
This material was summarized based on the above paper, and unauthorized use for commercial purposes is prohibited.
Copyright © 2025 CASTMAN. All rights reserved.