This introductory paper is the research content of the paper ["Developing a patient individualized flexible silicone implant using SLS and vacuum die casting"] published by [AIP Publishing].
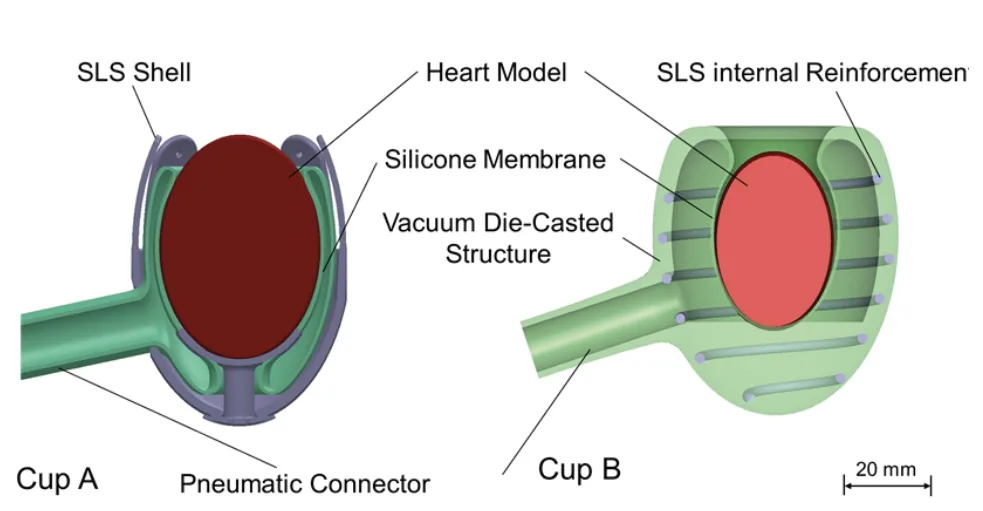
1. Overview:
- Title: Developing a patient individualized flexible silicone implant using SLS and vacuum die casting
- Author: Martin Launhardt, Nina Ebel, Markus Kondruweit, Michael Weyand, Tilmann Volk, Dietmar Drummer
- Publication Year: 2019
- Publishing Journal/Academic Society: AIP Conference Proceedings
- Keywords: SLS, Vacuum Die Casting, Silicone Implant, Heart Support, Patient Individualized, Flexible Implant
2. Abstracts / Introduction
In addressing the critical challenge of heart failure, which often necessitates heart transplantation, this study investigates the potential of internal flexible cardiac supporting devices. Such devices could serve as a bridge to transplantation or even obviate the need for it by enhancing cardiac function. The technical realization of this concept demands patient-individualized geometries, flexible structures, and strategic reinforcements to ensure compression forces are directed exclusively at the myocardium, sparing adjacent organs. Selective Laser Sintering (SLS) is explored for its capacity to fabricate complex thermoplastic components without conventional tooling. However, the limited material selection of SLS, particularly the absence of medical-grade silicone rubber, necessitates an indirect approach. This research analyzes the applicability of SLS-fabricated vacuum die casts for producing patient-specific flexible silicone implants. The study encompasses fundamental investigations into the molding and demolding of silicone specimens using SLS vacuum die casts, and the integration of flexible reinforcement structures. Initial mechanical assessments validate the manufacturing process and highlight optimization opportunities using patient-individualized geometries. The successful fabrication of functional silicone heart supporting structures, suitable for preliminary medical in-vitro evaluations, is demonstrated.
Cardiac insufficiency remains a prevalent and escalating health concern. While pharmacological interventions have advanced, heart transplantation remains the definitive treatment for end-stage chronic cardiac insufficiency. However, the scarcity of donor hearts limits its broad applicability, prompting the exploration of alternative therapeutic strategies. Heart supporting systems have emerged as a viable alternative to cardiac transplantation since the 1980s. Conventional systems, in direct contact with blood, pose risks of thromboembolism and infection. Consequently, research is directed towards extra-aortic systems that provide mechanical support through external cardiac compression, mitigating direct blood contact risks. However, the clinical translation of these systems is hindered by the lack of patient-individualized manufacturing capabilities, potentially leading to coronary vessel impingement. Selective Laser Sintering (SLS), an additive manufacturing technology for polymers, offers design freedom and complex part fabrication. While Polyamide 12 (PA12), a common SLS material, lacks medical certification for direct implantation, SLS can be indirectly employed to create vacuum die casts and investment castings for medical components using certified materials like liquid silicone. This paper investigates a manufacturing route combining SLS, vacuum die casting, investment casting, and dip coating to produce silicone-based heart supporting structures for initial small animal studies. The objective is to develop compact, internally reinforced, patient-specific systems that selectively apply pressure to the heart without affecting surrounding tissues. Preliminary mechanical tests are conducted to evaluate deformation and volumetric displacement of prototype implants.
3. Research Background:
Background of the Research Topic:
Heart failure represents a significant and growing medical challenge, often culminating in the necessity for heart transplantation. The limitations in donor organ availability necessitate the exploration of alternative therapeutic strategies, particularly for chronic cardiac insufficiency. Traditional heart supporting systems, which involve direct blood contact, carry inherent risks such as blood clot formation and infections. Therefore, externally applied cardiac support systems are under investigation to provide mechanical assistance to the heart without direct blood interaction. A critical challenge in this domain is the development of patient-individualized heart supporting structures to prevent potential impairment of coronary blood vessels, which are located on the epicardial surface.
Status of Existing Research:
Heart supporting systems have been explored as an alternative to cardiac transplantation since the 1980s. However, current extra-aortic heart supporting systems are not yet clinically implemented and lack patient-specific customization. This lack of individualization can lead to complications, particularly the potential constriction of coronary blood vessels.
Necessity of the Research:
The research is necessitated by the need for patient-individualized flexible heart supporting structures that can provide effective cardiac assistance without compromising coronary blood flow. Developing manufacturing methods capable of producing such customized implants is crucial for advancing the clinical applicability of extra-aortic heart support systems.
4. Research Purpose and Research Questions:
Research Purpose:
The primary research purpose is to investigate and validate feasible manufacturing methodologies for patient-individualized flexible heart supporting structures suitable for functional cardiac support systems. Specifically, the study aims to assess the applicability of utilizing SLS-generated vacuum die casts for the fabrication of patient-specific flexible silicone implants.
Key Research:
This research focuses on addressing the following key aspects:
- Exploration of two distinct manufacturing routes for creating patient-individualized heart supporting structures.
- Fundamental studies on the molding and demolding characteristics of silicone using SLS-produced vacuum die casts.
- Investigation into the integration of flexible reinforcement structures within the silicone implant design.
- Mechanical testing to evaluate the feasibility of the proposed manufacturing process and identify optimization opportunities for patient-specific geometries.
- Fabrication and functional assessment of initial silicone heart supporting structure prototypes for medical in-vitro evaluations.
5. Research Methodology
Research Design:
This research employs an experimental design to evaluate two distinct manufacturing methodologies for creating silicone heart implants: Cup A and Cup B. The study focuses on assessing the feasibility of each method through prototype fabrication and subsequent mechanical and volumetric performance testing.
Data Collection Method:
Data collection methods included:
- Visual Observation: Qualitative assessment of membrane contraction behavior during pneumatic actuation, as depicted in "FIGURE 3. Cup A during contraction without a heart shows a three folded silicone membrane shape".
- Optical Displacement Measurement: Quantitative assessment of cup expansion using optical methods, as illustrated in "FIGURE 4. Expansion test on cup B".
- Volumetric Displacement Measurement: Quantification of volumetric displacement towards the heart and outwards using a water-filled balloon dummy heart and a pressure device. Water height displacement and optical measurements were used to quantify volumetric changes.
Analysis Method:
The analysis involved a comparative assessment of the volumetric displacement and expansion characteristics of Cup A and Cup B variants, including those with different reinforcement configurations (circular helix, oval helix, and without helix). The volumetric displacement data, presented in "FIGURE 5. Volumetric displacement of cup B towards the outside divided into width and height" and "FIGURE 6. Volumetric displacement of cup A and B towards the heart", were analyzed to determine the efficacy of each design in directing pressure inwards towards the heart while minimizing outward expansion.
Research Subjects and Scope:
The research subjects were prototype silicone heart supporting structures, specifically Cup A and Cup B designs. The scope of the study was limited to the manufacturing feasibility assessment and basic functional testing of these prototypes. The prototypes were designed as initial iterations for potential future application in small animal in-vivo studies.
6. Main Research Results:
Key Research Results:
The study yielded several key findings:
- The combination of "SLS vacuum die casts with SLS lost core dip coating can be used for the manufacturing of complex silicone parts".
- "It was demonstrated that with defined SLS parameters lost cores and investment castings can be manufactured in order to realize complex geometries".
- "It was proven that it is possible to fully integrate a SLS reinforcement structure into the silicone".
- "Furthermore, the integration of an SLS structure provides a flexible structure and at the same time guides any expansion towards the heart".
- "Furthermore, it was shown, that the cup B with an internal helix reinforcement shows the least volumetric displacement on the outside and thus does not influence adjacent organs".
- "Furthermore, this cup also provides highest volumetric displacement on the inside of the cup and thus operates efficiently".
- Cup B with a circular profiled helix exhibited the highest volumetric displacement towards the heart, while Cup A demonstrated the lowest volumetric displacement.
Analysis of presented data:
- FIGURE 3: Demonstrates the contraction behavior of Cup A and Cup B during pneumatic actuation without a heart model. Cup A exhibits a "three folded silicone membrane shape", while Cup B shows a four-folded configuration.
- FIGURE 4: Illustrates the expansion test results for Cup B variants. Reinforced cups (circular and oval helix) show minimal expansion in width but some expansion in height. The cup without reinforcement exhibits the largest width expansion, up to 17%, potentially affecting adjacent organs.
- FIGURE 5 & 6: Quantify the volumetric displacement of Cup B towards the outside and Cup A and B towards the heart, respectively. Cup B with a circular helix reinforcement shows the highest volumetric displacement towards the heart and the lowest displacement outwards. Cup A shows the lowest volumetric displacement overall. The data suggests that internal SLS reinforcement in Cup B effectively directs energy inwards, enhancing efficiency.
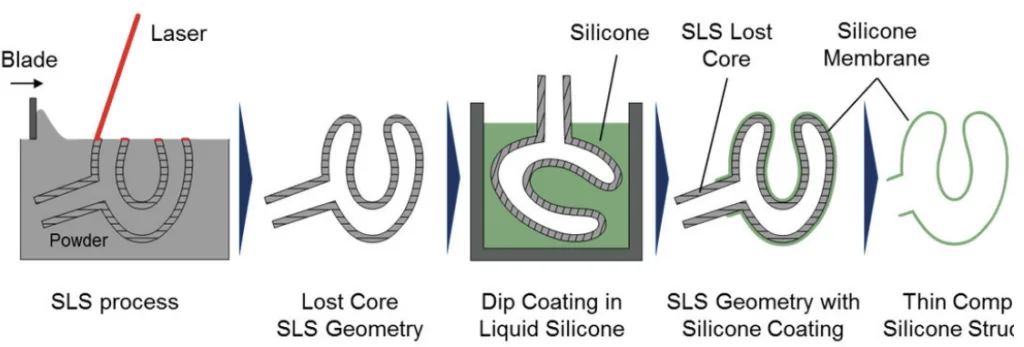
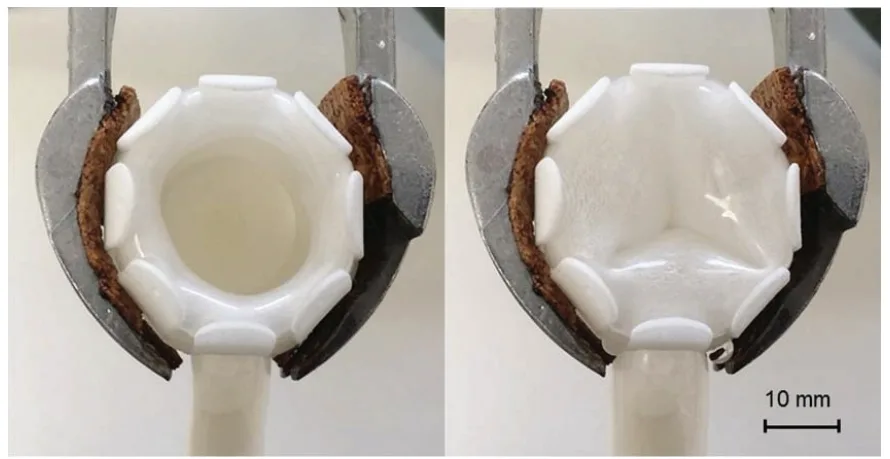
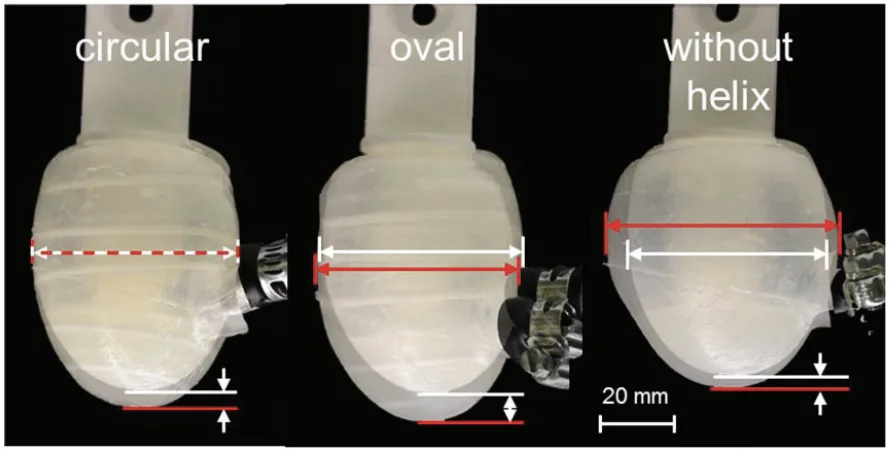
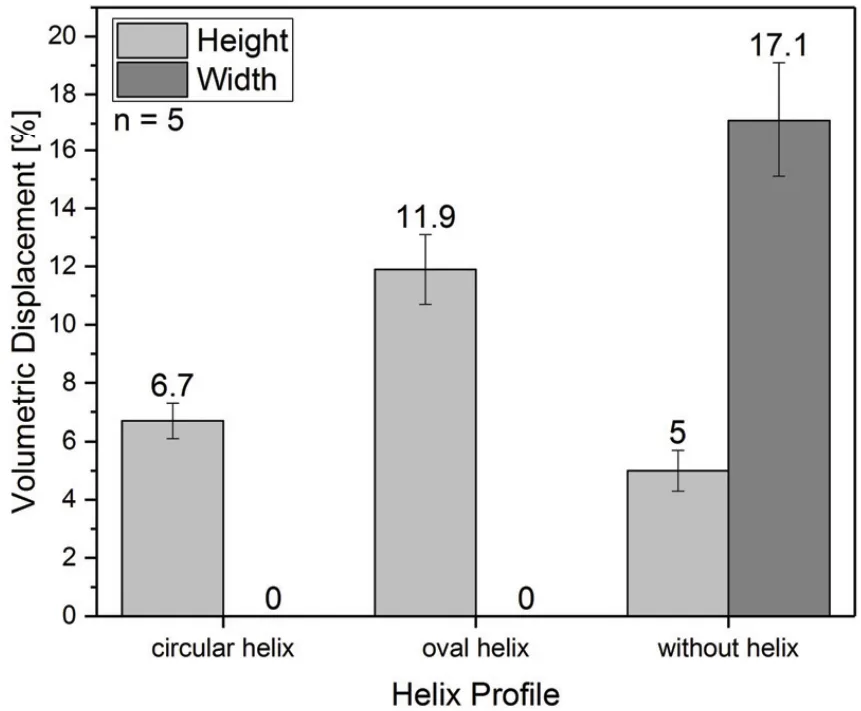
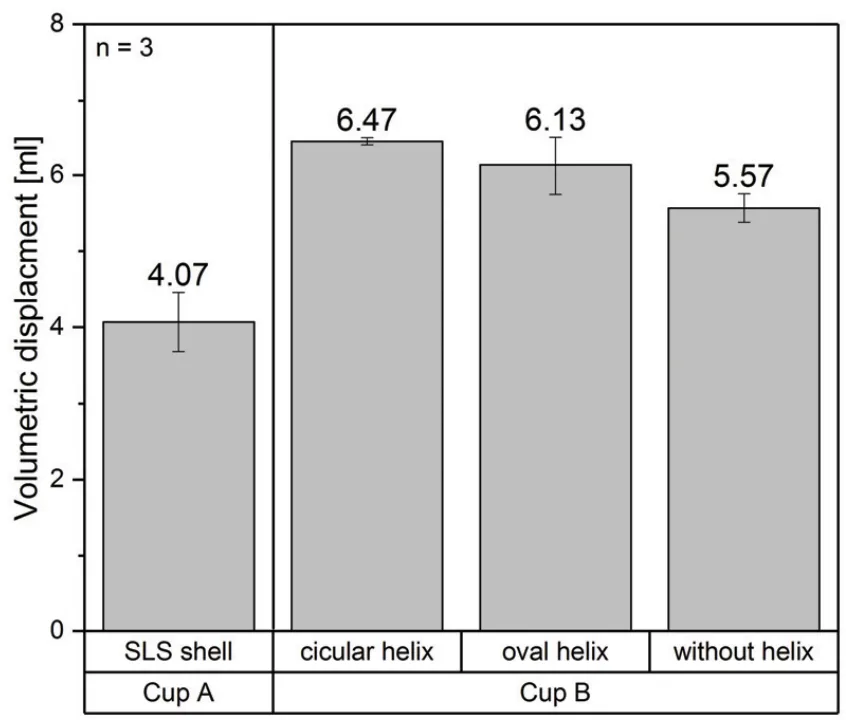
Figure Name List:
- FIGURE 1. Schematic of the cup designs A, (left) and B, (right)
- FIGURE 2. Schematic of the manufacturing of a complex silicone structure using SLS lost cores and dip coating process
- FIGURE 3. Cup A during contraction without a heart shows a three folded silicone membrane shape
- FIGURE 4. Expansion test on cup B
- FIGURE 5. Volumetric displacement of cup B towards the outside divided into width and height
- FIGURE 6. Volumetric displacement of cup A and B towards the heart
7. Conclusion:
Summary of Key Findings:
This research successfully demonstrated the feasibility of utilizing a combination of "SLS vacuum die casts with SLS lost core dip coating" for the fabrication of intricate silicone components essential for mechanical heart support systems. The study confirmed that "with defined SLS parameters lost cores and investment castings can be manufactured" to achieve complex geometries. Furthermore, the research proved the successful "integration of a SLS reinforcement structure into the silicone", which provides structural flexibility while effectively directing expansion forces towards the heart. Notably, "cup B with an internal helix reinforcement shows the least volumetric displacement on the outside" and the "highest volumetric displacement on the inside of the cup", indicating efficient and targeted cardiac support.
Academic Significance of the Study:
This study contributes to the field of medical device manufacturing by demonstrating the synergistic application of additive manufacturing (SLS) and conventional techniques (vacuum die casting, dip coating) for creating complex, patient-specific silicone implants. It provides a viable manufacturing pathway for developing customized heart supporting devices, potentially advancing the treatment of heart failure.
Practical Implications:
The developed manufacturing process and prototype designs have practical implications for the development of patient-individualized heart support systems. The successful fabrication of functional silicone heart supporting structures opens avenues for pre-clinical in-vitro testing and potential in-vivo evaluation in small animal models, paving the way for future clinical translation.
Limitations of the Study and Areas for Future Research:
The study acknowledges that "in future research the dynamic long time strength of the material and component needs to be tested". Further research should focus on evaluating the long-term durability and mechanical performance of the silicone implants. In-vitro and in-vivo studies are necessary to assess biocompatibility and functional efficacy in biological environments. Additionally, further investigation into the "impact of customized cup shapes" and optimization of reinforcement structures are warranted to enhance the performance and patient-specificity of these heart supporting systems.
8. References:
- 1. Statistisches Bundesamt, Die 10 häufigsten Todesfälle durch Herz-Kreislauf-Erkrankungen,
https://www.destatis.de/, accessed July 2017. - 2. S. J. Park, C. A. Milano, and A. J. Tatooles, et al., "Outcomes in advanced heart failure patients with left
ventricular assist devices for destination therapy,” Circulation. Heart Failure 5 (2), 241–248 (2012). - 3. H. Gorler and A. Haverich, “Herztransplantation und Assist Devices," Herz 29 (7), 695–701 (2004).
- 4. Deutsche Stiftung Organtransplantation, Informationen für den Organspendeprozess Koordinierung der
Organspende in Deutschland, https://www.dso.de/, accessed July 2017. - 5. U. Boeken, Mechanische Herz-Kreislauf-Unterstützung. Indikationen, Systeme, Implantationstechniken
(Springer, Berlin, 2013). - 6. M. Anstadt, G. Anstadt, and S. MacDonald, et al., Method and apparatus for direct mechanical ventricular
actuation with favorable conditioning and minimal heart stress, US20060211909 A1 . - 7. M. Struber, A. L. Meyer, and D. Malehsa, et al., “The current status of heart transplantation and the
development of "artificial heart systems",” Deutsches Ärzteblatt international 106 (28-29), 471–477 (2009). - 8. M. N. Kavarana, D. N. Helman, and M. R. Williams, et al., "Circulatory support with a direct cardiac
compression device,” The Journal of Thoracic and Cardiovascular Surgery 122 (4), 786–787 (2001). - 9. J. C. Criscione, S. Biswas, S. Hall, L. Harrison, and D. Robbins, Device for the modulation of cardiac end
diastolic volume (US7871366 B2) (2011).- E. T. Roche, M. A. Horvath, and I. Wamala, et al., “Soft robotic sleeve supports heart function," Science
translational medicine 9 (373) (2017).
- E. Erdmann, Klinische Kardiologie. Krankheiten des Herzens, des Kreislaufs und der herznahen Gefäße, 8.th
ed. (Springer, Berlin, 2011).
- C. Leiggener, E. Messo, and A. Thor, et al., “A selective laser sintering guide for transferring a virtual plan to
real time surgery in composite mandibular reconstruction with free fibula osseous flaps," International journal
of oral and maxillofacial surgery 38 (2), 187–192 (2009).
- J. Breuninger, R. Becker, and A. Wolf, et al., Generative Fertigung mit Kunststoffen. Konzeption und
Konstruktion für Selektives Lasersintern (Springer Verlag, Berlin, Heidelberg, 2013).
- M. Launhardt, M. Drexler, and D. Drummer, “Rapid-Manufacturing-Process for Elastomeric Components,"
International Journal of Recent Contributions from Engineering, Science & IT (iJES) 3 (2), 4–9 (2015).
- Datenblatt PolyConForm GmbH, Silikone PlatSilita 13-45 Н.
- E. T. Roche, M. A. Horvath, and I. Wamala, et al., “Soft robotic sleeve supports heart function," Science
9. Copyright:
- This material is "Martin Launhardt, Nina Ebel, Markus Kondruweit, Michael Weyand, Tilmann Volk, Dietmar Drummer"'s paper: Based on "Developing a patient individualized flexible silicone implant using SLS and vacuum die casting".
- Paper Source: https://doi.org/10.1063/1.5084908
This material was created to introduce the above paper, and unauthorized use for commercial purposes is prohibited.
Copyright © 2025 CASTMAN. All rights reserved.