This paper summary is based on the article Automotive Lightweight Design: Simulation Modeling of Mass-Related Consumption for Electric Vehicles presented at the MDPI
1. Overview:
- Title: Automotive Lightweight Design: Simulation Modeling of Mass-Related Consumption for Electric Vehicles
- Author: Francesco Del Pero, Lorenzo Berzi, Andrea Antonacci, and Massimo Delogu
- Publication Year: 2020
- Publishing Journal/Academic Society: Machines (MDPI)
- Keywords: lightweighting; mechanical design; industrial engineering; automotive; simulation modeling; energy consumption; sustainability
2. Research Background:
- Social/Academic Context of the Research Topic:
에너지 자원 고갈 및 지구 온난화는 현대 사회의 주요 관심사이며, 화석 연료 소비를 줄이기 위한 산업 및 연구 개발이 활발히 진행되고 있다 [1,2,3,4]. 도로 운송 부문은 전 세계 에너지 수요 및 대기 배출량의 상당 부분을 차지하며 [5], 자동차의 수명 주기 중 운행 단계가 가장 많은 에너지를 소비하므로 경량화 설계는 지속 가능한 이동성을 위한 매우 유망한 방법으로 부상하고 있다 [6]. 경량화는 자동차의 무게와 운행 중 에너지 소비가 밀접하게 관련되어 있어 사용 단계 소비를 줄이는 데 큰 잠재력을 제공한다. 10%의 무게 감소는 기능 수준을 유지하면서 약 3~6%의 연료 소비 감소로 이어진다 [10,11]. - Limitations of Existing Research:
기존 연구는 주로 내연 기관 자동차(ICEV)의 경량화에 초점을 맞추고 있으며 [32], 고급 파워트레인 차량(전기, 하이브리드, 연료 전지)에 대한 연구는 제한적이다 [38,39]. 특히, 배터리 전기 자동차(BEV)의 경우, 연료-질량 상관관계가 ICEV에 비해 낮게 나타나며, 이는 BEV의 높은 파워트레인 효율과 낮은 에너지 소비 때문으로 분석된다 [43]. Kim and Wallington [44]의 연구는 BEV를 포함한 다양한 파워트레인 기술에 대한 FRV를 조사한 유일한 연구이지만, 특정 자동차 모델에 기반한 연구이며, 실제 차량에 대한 질량-소비 상관관계 추정에 대한 지침을 제공하지 않는다. 또한, 기존 연구들은 주로 이론적인 자동차 모델을 기반으로 시뮬레이션 모델링을 수행하며, 실제 자동차 모델명 및 모델 연도에 대한 정보가 부족하고, 특정 지역의 표준화된 주행 사이클에 기반하여 계산되어 전 세계적인 일반성을 확보하기 어렵다는 한계가 있다. - Necessity of the Research:
기존 연구의 한계점을 극복하고 BEV 경량화의 에너지 및 지속 가능성 영향을 정확하게 평가하기 위해 실제 자동차 모델 데이터를 기반으로 질량 유도 에너지 소비를 분석하는 연구가 필요하다. 특히, 다양한 주행 패턴을 고려하고, 환경 영향 감소 가치(IRV)를 포함하여 지속 가능성 평가를 수행하는 연구가 요구된다.
3. Research Purpose and Research Questions:
- Research Purpose:
본 연구의 목표는 순수 전기 자동차의 질량 유도 에너지 소비에 대한 분석적 계산 절차를 제시하고, BEV 분야 내 경량화의 에너지 및 지속 가능성 영향 평가를 지원하는 것이다. - Key Research Questions:
- 다양한 BEV 모델 및 주행 조건에서 에너지 감소 가치(ERV)는 어떻게 변화하는가?
- 경량화 설계가 환경에 미치는 영향은 전력망 구성에 따라 어떻게 달라지는가?
- 실제 자동차 모델의 기술적 특징을 고려하여 ERV를 정확하게 추정할 수 있는 모델링 접근 방식은 무엇인가?
- Research Hypotheses:
- ERV는 차량 크기, 주행 사이클, 전력망 구성에 따라 크게 달라질 것이다.
- 차량 질량, 최대 출력, 출력-질량비와 같은 기술적 특징은 ERV에 영향을 미칠 것이다.
- 실제 차량 데이터를 기반으로 한 분석적 모델은 BEV 경량화의 에너지 및 환경적 이점을 보다 정확하게 평가할 수 있도록 할 것이다.
4. Research Methodology
- Research Design:
본 연구는 분석적 계산 절차를 기반으로 한 시뮬레이션 모델링 접근 방식을 채택하였다. MATLAB-Simulink 소프트웨어를 사용하여 차량 동역학 시뮬레이션 모델을 개발하고, 다양한 BEV 모델 및 주행 조건에서 에너지 소비량을 계산하였다. - Data Collection Method:
2019년 유럽 시장에서 판매되는 실제 BEV 모델의 기술적 특징 데이터를 수집하여 사례 연구에 활용하였다. 에너지 소비량 추정을 위해 NEDC, WLTP, ALDC의 세 가지 주행 사이클을 사용하였다. - Analysis Method:
차량 질량 변화에 따른 에너지 소비량 변화를 분석하기 위해 에너지 감소 가치(ERV) 계수를 계산하였다. ERV는 100kg 질량 감소를 통해 달성할 수 있는 특정 소비 절감량을 정량화한다. 또한, 환경 영향 감소 가치(IRV)를 계산하여 경량화 설계의 환경적 영향을 평가하였다. IRV는 세 가지 전력망 구성(노르웨이, 유럽 평균, 폴란드)에 대해 추정되었다. - Research Subjects and Scope:
A/B, C, D/E 클래스에 속하는 10가지 BEV 모델을 사례 연구 대상으로 선정하였다. 다양한 차량 크기를 포괄함으로써 질량과 전기 흡수 사이의 상관관계를 모델링하고 차량 기술의 강력한 변화를 고려할 수 있도록 하였다.
5. Main Research Results:
- Key Research Results:
- ERV는 차량 모델 및 주행 사이클에 따라 0.47–1.17 kWh/(100 km × 100 kg) 범위에서 넓게 변동하며, 주로 차량 크기와 주행 사이클에 따라 변동성이 나타난다.
- ALDC 주행 사이클은 NEDC 및 WLTP보다 높은 질량 유도 소비(ERVALDC)를 제공하며, 이는 ALDC의 역동적인 주행 특성 때문으로 분석된다.
- ERV는 차량 크기가 증가함에 따라 증가하며, 이는 에너지 수요가 높은 대형 차량일수록 경량화 효과가 더 크다는 것을 의미한다.
- IRV는 전력망 구성에 따라 크게 달라지며, 노르웨이 전력망(NO)은 가장 낮은 IRV를, 폴란드 전력망(PL)은 가장 높은 IRV를 나타낸다.
- 차량 질량(M)은 ERV와 가장 강력한 상관관계를 가지며, 최대 출력(Pmax) 및 출력-질량비(P/M)보다 높은 상관계수(R2)를 보인다.
- Statistical/Qualitative Analysis Results:
- Table 1은 모든 차량 사례 연구 및 주행 사이클에 대한 ERV 및 IRV 계수를 보고한다.
- Table 2는 최소값, 최대값, 범위, 산술 평균 및 표준 편차 측면에서 자동차 클래스/주행 사이클별 ERV/IRV 분석을 보여준다.
- Figure 4는 차량 클래스 및 주행 사이클별 사례 연구에 대한 ERV의 산술 평균을 보여준다.
- Figure 5는 전기 소비량의 함수로서 모든 사례 연구에 대한 ERV를 보여준다.
- Figure 6은 차량 클래스 및 주행 사이클별 사례 연구에 대한 IRV의 산술 평균을 보여준다.
- Figure 7은 주요 차량 기술 특징의 함수로서 모든 사례 연구에 대한 ERV를 보여준다: 회귀선. 차량 질량(M) (a), 최대 출력 (Pmax) (b), 출력-질량비 (P/M) (c).
- Figure 8은 차량 질량의 함수로서 모든 사례 연구에 대한 IRV를 보여준다: 회귀선.
- Figure 9는 지속 가능성 사례 연구(전면 모듈(FM), 전면 후드(FH), 전면 도어(FD), 크래시 대시보드 빔(CDB), 서스펜션 암(SA))에 대한 손익분기점(BEP)을 보여준다.
- Figure 10은 지속 가능성 사례 연구 FM, FH 및 SA에 대한 자동차 질량의 함수로서 BEP를 보여준다. 노르웨이 그리드 믹스(a), 유럽 평균 그리드 믹스(b), 폴란드 그리드 믹스(c).
- Data Interpretation:
- ERV는 차량 크기, 주행 조건, 전력망 구성 등 다양한 요인에 따라 크게 달라지므로, 경량화 설계의 효과를 평가할 때 이러한 요인들을 종합적으로 고려해야 한다.
- ALDC와 같이 역동적인 주행 조건에서는 경량화의 에너지 절감 효과가 더욱 두드러지게 나타난다.
- 전력망의 탄소 집약도가 높을수록 경량화의 환경적 이점은 더욱 커진다.
- 차량 질량은 ERV를 예측하는 데 가장 중요한 기술적 특징이며, 차량 무게 정보를 이용하여 ERV를 효과적으로 추정할 수 있다.
- Figure Name List:
- Figure 1. Layout of MATLAB-Simulink model: driver (a), powertrain (b), driveline (c), and energy management (d).
- Figure 2. Look-up-tables for basic motor characteristics.
- Figure 3. Brake-blending criteria: typical braking repartition in absence of regenerative braking capabilities (a) and brake blending for vehicles capable of regenerative braking capabilities on front axle (b).
- Figure 4. Arithmetic mean of ERV over case studies per vehicle class and driving cycle.
- Figure 5. ERV for all case studies in function of electricity consumption.
- Figure 6. Arithmetic mean of IRV over case studies per vehicle class and driving cycle.
- Figure 7. ERV for all case studies in function of main vehicle technical features: regression lines. Vehicle mass (M) (a), maximum power (Pmax) (b), and power-to-mass ratio (P/M) (c).
- Figure 8. IRV for all case studies in function of vehicle mass: regression lines.
- Figure 9. Break-Even Point (BEP) for sustainability case studies (Front Module (FM), Front Hood (FH), Front Door (FD), Crash Dashboard Beam (CDB), and Suspension Arm (SA)).
- Figure 10. BEP in function of car mass for sustainability case studies FM, FH, and SA. Norwegian grid mix (a), average European grid mix (b), Polish grid mix (c).
- Figure A1. Speed profile of the ALDC.
- Figure A2. Energy consumption in function of mass with regression lines and ERV coefficient.
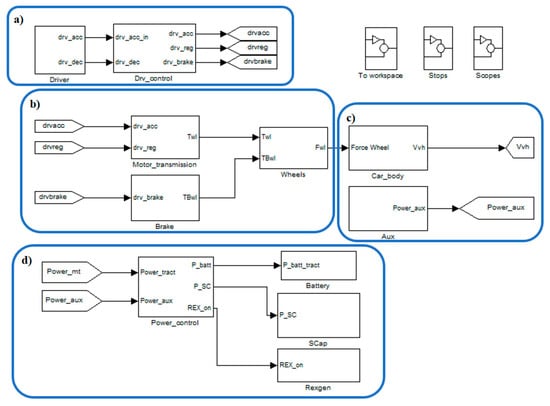
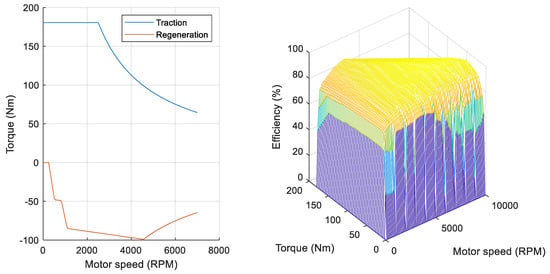
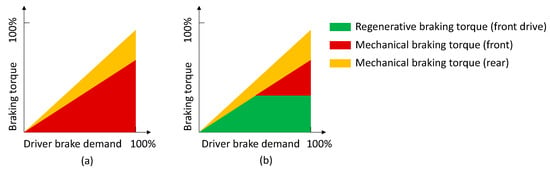
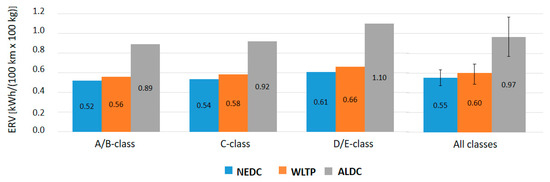
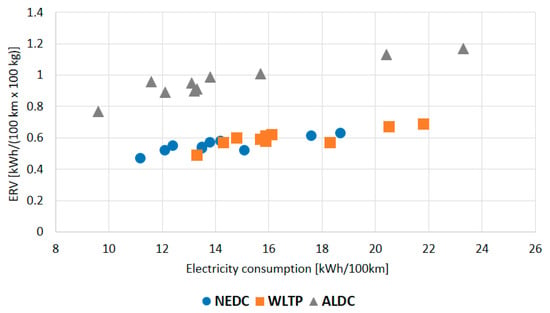
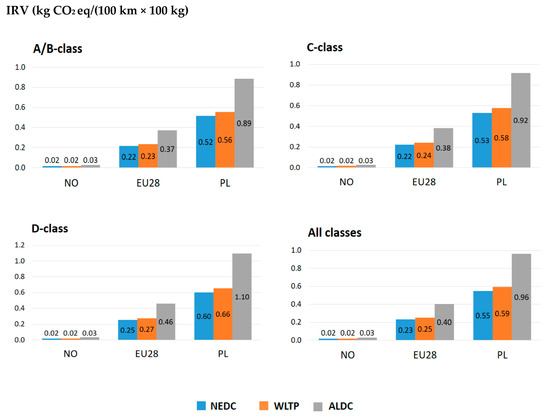
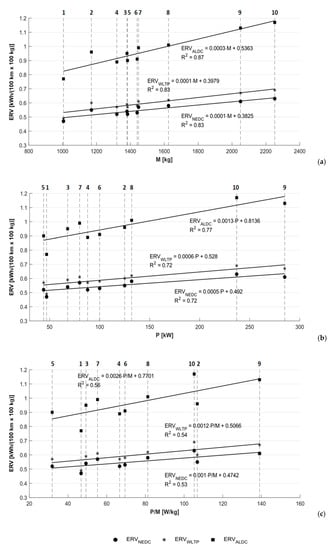
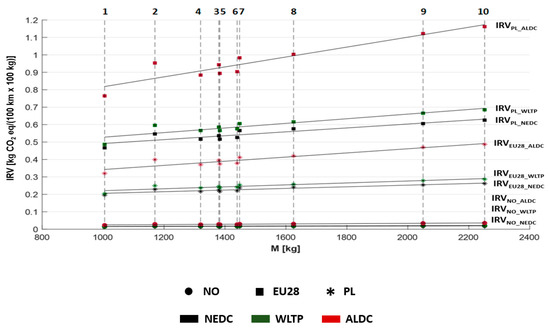
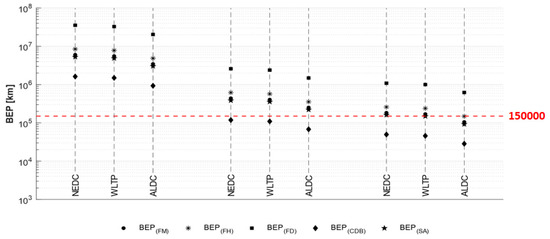
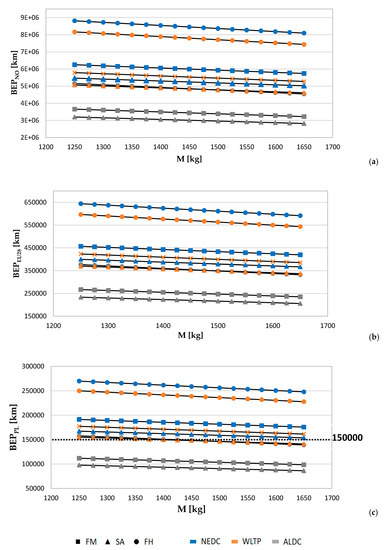
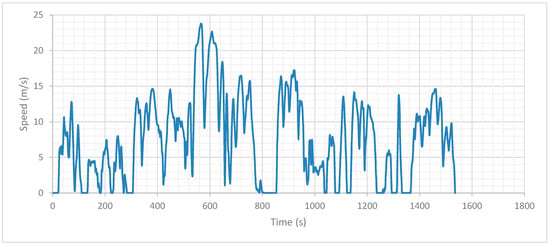
6. Conclusion and Discussion:
- Summary of Main Results:
본 연구는 BEV의 질량 유도 에너지 소비를 평가하기 위한 분석적 프레임워크를 개발하고, 실제 차량 모델 및 다양한 주행 조건을 고려하여 ERV 및 IRV 계수를 추정하였다. 연구 결과, ERV는 차량 모델, 주행 사이클, 전력망 구성에 따라 크게 달라지며, 차량 질량이 ERV에 가장 큰 영향을 미치는 요인임을 확인하였다. 또한, 경량화 설계의 환경적 이점은 전력망의 탄소 집약도에 따라 크게 달라질 수 있음을 보여주었다. - Academic Significance of the Research: 본 연구는 BEV 경량화 연구 분야에 다음과 같은 학문적 의의를 가진다.
- BEV의 질량 유도 에너지 소비에 대한 심층적인 분석 및 데이터 제공
- 실제 차량 모델 데이터를 기반으로 한 ERV/IRV 추정 방법론 제시
- 다양한 주행 조건 및 전력망 구성을 고려한 경량화 효과 분석 프레임워크 개발
- Practical Implications: 본 연구 결과는 자동차 산업계 및 정책 입안자에게 다음과 같은 실질적인 시사점을 제공한다.
- BEV 경량화 설계의 에너지 및 환경적 이점을 정량적으로 평가하는 데 활용 가능
- 차량 모델, 주행 조건, 전력망 구성을 고려한 맞춤형 경량화 전략 수립 지원
- 경량화 기술 개발 및 보급 정책 수립의 기초 자료 제공
- Limitations of the Research: 본 연구는 다음과 같은 제한점을 가진다.
- 시뮬레이션 모델은 실제 차량의 복잡성을 완벽하게 반영하지 못할 수 있다.
- 사례 연구는 특정 BEV 모델 및 주행 조건에 한정되어 있다.
- 전력망 구성은 세 가지 대표적인 시나리오만 고려되었다.
7. Future Follow-up Research:
- Directions for Follow-up Research: 향후 연구는 다음과 같은 방향으로 진행될 수 있다.
- 보다 다양한 BEV 모델 및 파워트레인 기술에 대한 연구 확대
- 실제 도로 주행 데이터를 기반으로 한 모델 검증 및 개선
- 차량 경량화 기술의 비용 및 경제성 분석 포함
- 전력망 구성 변화 및 재생 에너지 보급 확대에 따른 경량화 효과 변화 연구
- Areas Requiring Further Exploration:
- 차량 경량화가 차량 성능, 안전성, 승차감 등 다른 차량 특성에 미치는 영향
- 경량화 기술의 수명 주기 평가(LCA) 및 전 과정 환경 영향 분석
- 소비자 수용성 및 시장성 분석을 통한 경량화 기술 보급 전략 연구
8. References:
- [1] Intergovernmental Panel on Climate Change. Climate Change 2014: Impacts, Adaptation, and Vulnerability; Cambridge University Press: Cambridge, UK; New York, NY, USA, 2014; Volume 1.
- [2] U.S. Environmental Protection Agency. DRAFT Inventory of U.S. Greenhouse Gas. Emissions and Sinks: 1990-2016; U.S. Environmental Protection Agency: Washington, DC, USA, 2018.
- [3] Intergovernmental Panel on Climate Change. Climate Change 2013: The Physical Science Basis, Working Group I Contribution to the Fifth Assessment; Cambridge University Press: Cambridge, UK, 2013.
- [4] Mashayekh, Y.; Jaramillo, P.; Samaras, C.; Hendrickson, C.T.; Blackhurst, M.; MacLean, H.L.; Matthews, H.S. Potentials for sustainable transportation in cities to alleviate climate change impacts. Environ. Sci. Technol. 2012, 46, 2529-2537. [CrossRef] [PubMed]
- [5] Milovanoff, A.; Kim, H.C.; De Kleine, R.; Wallington, T.J.; Posen, I.D.; MacLean, H.L. A Dynamic Fleet Model of U.S Light-Duty Vehicle Lightweighting and Associated Greenhouse Gas Emissions from 2016 to 2050. Environ. Sci. Technol. 2019, 53, 2199-2208. [CrossRef] [PubMed]
- [6] Gonzalez Palencia, J. Energy use and CO2 emissions reduction potential in passenger car fleet using zero emission vehicles and lightweight materials. Energy 2012, 48, 548-565. [CrossRef]
- [7] Tisza, M.; Czinege, I. Comparative study of the application of steels and aluminium in lightweight production of automotive parts. Int. J. Lightweight Mater. Manuf. 2018, 1, 229-238. [CrossRef]
- [8] Das, S.; Graziano, D.; Upadhyayula, V.K.K.; Masanet, E.; Riddle, M.; Cresko, J. Vehicle lightweighting energy use impacts in U.S. light-duty vehicle fleet. Sustain. Mater. Technol. 2016, 8, 5-13. [CrossRef]
- [9] Luk, J.; Kim, H.C.; De Kleine, R.; Wallington, T.J.; MacLean, H. Impact of powertrain type on potential life cycle greenhouse gas emission reduction from a real world lightweight glider. SAE Tech. Pap. Ser. 2017, 1-8. [CrossRef]
- [10] Modaresi, R.; Pauliuk, S.; Løvik, A.N.; Muller, D.B. Global Carbon Benefits of Material Substitution in Passenger Cars until 2050 and the Impact on the Steel and Aluminum Industries. Environ. Sci. Technol. 2014, 48, 10776-10784. [CrossRef]
- [11] Koffler, C.; Rodhe-Branderburger, K. On the calculation of fuel savings through lightweight design in automotive life cycle assessments. Int. J. Life Cycle Assess. 2010, 15, 128–135. [CrossRef]
- [12] Sah, S.; Bawase, M.; Saraf, M. Light-weight Materials and their Automotive Applications. SAE Tech. Pap. 2014, 28, 25. [CrossRef]
- [13] Ferreira, V. Lightweight automotive components based on nano-diamond-reinforced aluminium alloy: A technical and environmental evaluation. Diam. Relat. Mater. 2019, 2019, 92.
- [14] Mayyas, A.T.; Qattawi, A.; Mayyas, A.R.; Omar, M.A. Life cycle assessment based selection for a sustainable lightweight body-in-white design. Energy 2012, 39, 412-425. [CrossRef]
- [15] Poulikidou, S.; Jerpdal, L.; Bjorklund, A.; Åkermo, M. Environmental performance of self-reinforced composites in automotive applications. Case study on a heavy truck component. Mater. Des. 2016, 103, 321-329. [CrossRef]
- [16] Kim, H.C.; Wallington, T.J. Life-Cycle Energy and Greenhouse Gas Emission Benefits of Lightweighting in Automobiles: Review and Harmonization. Environ. Sci. Technol. 2013, 47, 6089-6097. [CrossRef]
- [17] Lewis, A.M.; Kelly, J.C.; Keoleian, G.A. Vehicle lightweighting vs. electrification: Life cycle energy and GHG emissions results for diverse powertrain vehicles. Appl. Energy 2014, 126, 13–20. [CrossRef]
- [18] Egede, P. Environmental Assessment of Lightweight Electric Vehicles; Springer International Publishing: Cham, Switzerland, 2017.
- [19] Alonso, E.; Lee, T.M.; Bjelkengren, C.; Roth, R.; Kirchain, R.E. Evaluating the Potential for Secondary Mass Savings in Vehicle Lightweighting. Environ. Sci. Technol. 2012, 46, 2893–2901. [CrossRef] [PubMed]
- [20] Egede, P. Sustainable Production, Life Cycle Engineering and Management. In Environmental Assessment of Lightweight Electric Vehicles, 1st ed.; Springer International Publishing: Cham, Switzerland, 2016.
- [21] Vinodh, S.; Jayakrishna, K. Environmental impact minimisation in an automotive component using alternative materials and manufacturing processes. Mater. Des. 2011, 32, 5082-5090. [CrossRef]
- [22] Raugei, M.; Morrey, D.; Hutchinson, A.; Winfield, P. A coherent life cycle assessment of a range of lightweighting strategies for compact vehicles. J. Clean. Prod. 2015, 108, 1168–1176. [CrossRef]
- [23] Weimar, E.; Finkbeiner, M. Statistical analysis of empirical lifetime mileage data for automotive LCA. Int. J. Life Cycle Assess. 2016, 21, 215-223. [CrossRef]
- [24] Bian, J.; Mohrbacher, H.; Zhang, J.S.; Zhao, Y.T.; Lu, H.Z.; Dong, H. Application potential of high performance steels for weight reduction and efficiency increase in commercial vehicles. Adv. Manuf. 2015, 3, 27–36. [CrossRef]
- [25] Faßbender, S.; Broöckerhoff, D.E.; Eckstein, L.; Hartmann, B.; Urban, P. Investigation of the Trade-Off between Lightweight and Battery Cost for an Aluminum-Intensive Electric Vehicle; Report 106330; Forschungsgesellschaft Kraftfahrwesen mbH Aachen: Aachen, Germany, 2012.
- [26] Schuh, G.; Korthals, K.; Backs, M. Environmental Impact of Body Lightweight Design in the Operating Phase of Electric Vehicles. In Proceedings of the 20th CIRP International Conference on Life Cycle Engineering, Singapore, 17-19 April 2013; Springer: Singapore.
- [27] Wilhelm, E.; Hofer, J.; Schenler, W.; Guzzella, L. Optimal implementation of lightweighting and powertrain efficiency in passengers' vehicles. Transport 2012, 27, 237–249. [CrossRef]
- [28] Pagerit, S.; Sharer, P.; Rousseau, A. Fuel Economy Sensitivity to Vehicle Mass for Advanced Vehicle Powertrains. SAE Tech. Pap. 2006, 1, 665.
- [29] Ridge, L. EUCAR-Automotive LCA Guidelines—Phase 2. SAE Tech. Pap. 1998. [CrossRef]
- [30] United States Environmental Protection Agency. New Fuel Economy and Environment Labels for a New Generation of Vehicles; EPA-420-F-11-017; United States Environmental Protection Agency: Washington, DC, USA, 2011.
- [31] Muttana, S.B.; Sardar, A. Lightweighting of Battery Electric Cars: An Impact Analysis Using Indian Driving Cycle. SAE Tech. Pap. Ser. 2013. [CrossRef]
- [32] Kim, H.C.; Wallington, T.J.; Sullivan, J.L.; Keoleian, G.A. Life cycle assessment of vehicle lightweighting: Novel mathematical methods to estimate use-phase fuel consumption. Environ. Sci. Technol. 2015, 49, 10209-10216. [CrossRef]
- [33] Kelly, J.C.; Sullivan, J.L.; Burnham, A.; Elgowainy, A. Impacts of vehicle weight reduction via material substitution on life-cycle greenhouse gas emissions. Environ. Sci. Technol. 2015, 49, 12535–12542. [CrossRef]
- [34] Hofer, J.; Wilhelm, E.; Schenler, W. Comparing the mass, energy, and cost effects of lightweighting in conventional and electric passenger vehicles. J. Sustain. Dev. Energy Water Environ. Syst. 2014, 2, 284–295. [CrossRef]
- [35] Del Pero, F.; Delogu, M.; Pierini, M. The effect of lightweighting in automotive LCA perspective: Estimation of mass-induced fuel consumption reduction for gasoline turbocharged vehicles. J. Clean. Prod. 2017, 154, 566-577. [CrossRef]
- [36] Delogu, M.; Del Pero, F.; Pierini, M. Lightweight design solutions in the automotive field: Environmental modelling based on Fuel Reduction Value applied to diesel turbocharged vehicles. Sustainability 2016, 8, 1167. [CrossRef]
- [37] Kim, H.C.; Wallington, T.J. Life cycle assessment of vehicle lightweighting: A physics-based model of mass-induced fuel consumption. Environ. Sci. Technol. 2013, 47, 14358–14366. [CrossRef]
- [38] An, F.; Santini, D.J. Mass Impacts on Fuel Economies of Conventional vs. Hybrid Electric Vehicles. In Proceedings of the SAE World Congress, Detroit, MI, USA, 8–11 March 2004.
- [39] Redelbach, M.; Klotzke, M.; Friedrich, H.E. Impact of Lightweight Design on Energy Consumption and Cost Effectiveness of Alternative Powertrain Concepts. In Proceedings of the European Vehicle Congress (EEVC), Brussel, Belgium, 19–22 November 2012.
- [40] Casadei, A.; Broda, R. Impact of Vehicle Weight Reduction on Fuel Economy for Various Vehicle Architectures; Project FB769; Ricardo Inc.: Shoreham-by-Sea, UK, 2008.
- [41] Wohlecker, R.; Johannaber, M.; Espig, M. Determination of Weight Elasticity of Fuel Economy for ICE, Hybrid and Fuel Cell Vehicles. SAE Tech. Pap. 2007, 1, 343.
- [42] U.S. Environmental Protection Agency. Light-Duty Automotive Technology, Carbon Dioxide Emissions, and Fuel Economy Trends: 1975 through 2016; U.S. Environmental Protection Agency: Washington, DC, USA, 2016.
- [43] Carlson, R.; Lohse-Busch, H.; Diez, J.; Gibbs, J. The measured impact of vehicle mass on road load forces and energy consumption for a BEV, HEV and ICE vehicle. SAE Int. J. Altern. Powertrains 2013, 2, 105–114. [CrossRef]
- [44] Kim, H.C.; Wallington, T.J. Life Cycle Assessment of Vehicle Lightweighting: A Physics-Based Model to Estimate Use-Phase Fuel Consumption of Electrified Vehicles. Environ. Sci. Technol. 2016, 50, 11226–11233. [CrossRef] [PubMed]
- [45] Luk, J.M.; Kim, H.C.; De Kleine, R.; Wallington, T.J.; MacLean, H.L. Review of the Fuel Saving, Life Cycle GHG Emission, and Ownership Cost Impacts of Lightweighting Vehicles with Different Powertrains. Environ. Sci. Technol. 2017, 51, 8215-8228. [CrossRef] [PubMed]
- [46] Mathworks. Available online: https://it.mathworks.com (accessed on 5 July 2020).
- [47] Argonne National Laboratory. GREET 2015 Model. In Transportation Research and Analysis Computing Center; Argonne National Laboratory: Argonne, IL, USA, 2015.
- [48] Berzi, L.; Delogu, M.; Pierini, M. A comparison of electric vehicles use-case scenarios: Application of a simulation framework to vehicle design optimization and energy consumption assessment. In Proceedings of the 2016 IEEE 16th International Conference on Environment and Electrical Engineering (EEEIC), Florence, Italy, 7-10 June 2016; pp. 1–6. [CrossRef]
- [49] National Renewable Energy Laboratory Future Automotive Systems Technology Simulator (FASTSim). Available online: http://www.nrel.gov/transportation/fastsim.html (accessed on 10 July 2020).
- [50] Burress, T.; Campbell, S. Benchmarking EV and HEV power electronics and electric machines. In Proceedings of the 2013 IEEE Transportation Electrification Conference and Expo (ITEC), Dearborn, MI, USA, 16–19 June 2013; pp. 1-6. [CrossRef]
- [51] Barlow, T.J.; Latham, S.; McCrae, I.S.; Boulter, P.G. A Reference Book of Driving Cycles for Use in the Measurement of Road Vehicle Emissions; TRL: Berks, UK, 2009.
- [52] Marotta, A.; Tutuianu, M. Europe-centric light duty test cycle and differences with respect to the WLTP cycle. In European Commission—Joint Research Centre—Scientific and Policy Reports; Publications Office of the European Union: Brussels, Belgium, 2012.
- [53] ASTERICS Project. Available online: https://egvi.eu/research-project/asterics/ (accessed on 15 July 2020).
- [54] Berzi, L.; Delogu, M.; Pierini, M. Development of driving cycles for electric vehicles in the context of the city of Florence. Transp. Res. Part D Transp. Environ. 2016, 47, 299-322. [CrossRef]
- [55] World Auto Steel. Future Steel Vehicle Overview Report; World Auto Steel: Brussels, Belgium, 2011.
- [56] Cubito, C.; Millo, F.; Boccardo, G.; Di Pierro, G.; Ciuffo, B.; Fontaras, G.; Serra, S.; Otura Garcia, M.; Trentadue, G. Impact of Different Driving Cycles and Operating Conditions on CO2 Emissions and Energy Management Strategies of a Euro-6 Hybrid Electric Vehicle. Energies 2017, 10, 1590. [CrossRef]
- [57] Weiss, M.B.P.; Hummel, R.; Steininger, N. A complementary emissions test for light-Duty vehicels: Assessing the technical feasibility of candidate procedures. JRC Sci. Policy Rep. 2013. [CrossRef]
- [58] Mock, P.; Kulhwein, J.; Tietge, U.; Franco, V.; Bandivadekar, A.; German, J. The WLTP: How a New Test Procedure for Cars will Affect Fuel Consumption Values in the EU; Working paper; The International Council on Clean Transportation (ICCT): Washington, DC, USA, 2014.
- [59] Fontaras, G.; Ciuffo, B.; Zacharofa, N.; Tsiakmakisa, S.; Marotta, S.; Pavlovica, J.; Anagnostopoulosa, K. The difference between reported and real-world CO2 emissions: How much improvement can be expected by WLTP introduction? Transp. Res. Procedia 2017, 25, 3933-3943. [CrossRef]
- [60] Delogu, M.; Zanchi, L.; Dattilo, C.A.; Pierini, M. Innovative composites and hybrid materials for electric vehicles lightweight design in a sustainability perspective. Mater. Today Commun. 2017, 13, 192–209. [CrossRef]
9. Copyright:
- This material is "Francesco Del Pero, Lorenzo Berzi, Andrea Antonacci, and Massimo Delogu"'s paper: Based on "Automotive Lightweight Design: Simulation Modeling of Mass-Related Consumption for Electric Vehicles".
- Paper Source: https://doi.org/10.3390/machines8030051
This material was summarized based on the above paper, and unauthorized use for commercial purposes is prohibited.
Copyright © 2025 CASTMAN. All rights reserved.
자동차 경량 설계: 전기 자동차의 대량 관련 소비 시뮬레이션 모델링
by Francesco Del Pero *,Lorenzo Berzi,Andrea Antonacci andMassimo DeloguDepartment of Industrial Engineering, University of Florence, Via di S. Marta 3, 50139 Florence, Italy*Author to whom correspondence should be addressed.Machines2020, 8(3), 51; https://doi.org/10.3390/machines8030051Received: 14 August 2020 / Revised: 30 August 2020 / Accepted: 31 August 2020 / Published: 3 September 2020
Abstract
차량 경량화와 관련된 수명 주기 효과에 대한 철저한 평가는 사용 단계에서 에너지 및 지속 가능성 이점이 직접적으로 의존하는 대량 유도 소비에 대한 엄격한 평가가 필요합니다. 기존 문헌은 이 연구 분야에 대해 상당한 개선 여지가 있기 때문에 이 논문은 순수 전기 자동차(EV)의 중량 관련 에너지 소비를 추정하기 위한 분석적 계산 절차를 제안합니다.
소비량과 질량 간의 상관 관계는 100kg 질량 감소를 통해 달성할 수 있는 특정 소비량 절감을 수량화하는 에너지 감소 값(ERV) 계수를 통해 표현됩니다. ERV는 실제 2019 유럽 시장 EV 모델에서 파생된 여러 이질적인 사례 연구에 대해 추정되며 3가지 주행 주기에 따라 서로 다른 운전 행동을 고려합니다.
고려 중인 사례 연구의 경우 ERV 범위는 0.47~1.17kWh/(100km × 100kg)이며 변동성은 주로 차량 크기와 주행 주기에 따라 다릅니다. 자동차 크기에 대한 질량 관련 소비의 높은 불확실성을 감안할 때, 차량 기술적 특징에서 시작하여 실제 EV 모델에 대한 ERV를 정확하게 추정하기 위해 분석 방법이 개선되었습니다.
에너지 평가와 함께 이 연구는 또한 세 가지 다른 전력 그리드 믹스에 대해 추정되는 충격 감소 값(IRV)을 통해 경량 설계의 환경적 영향을 평가합니다. 마지막으로 ERV/IRV 모델링 접근 방식은 문헌에서 가져온 일련의 비교 경량 사례 연구에 적용됩니다.
키워드: 경량화 ; 기계 설계 ; 산업 공학 ; 자동차 ; 시뮬레이션 모델링 ; 에너지 소비 ; 지속 가능성