1. 개요:
- 제목: 고압 다이캐스팅 마그네슘-알루미늄 기반 합금의 기계적 성질 및 변형 거동
- 저자: 화첸 앙 (Hua Qian Ang, Vivian)
- 발행 연도: 2017년 10월
- 발행 저널/학회: RMIT 대학교 공학대학원, 과학 공학 및 보건 대학 박사 학위 논문
- 키워드: 고압 다이캐스팅; 마그네슘 합금; 변형 거동; 기계적 성질; 변형률 속도 민감도; 구성 모델링
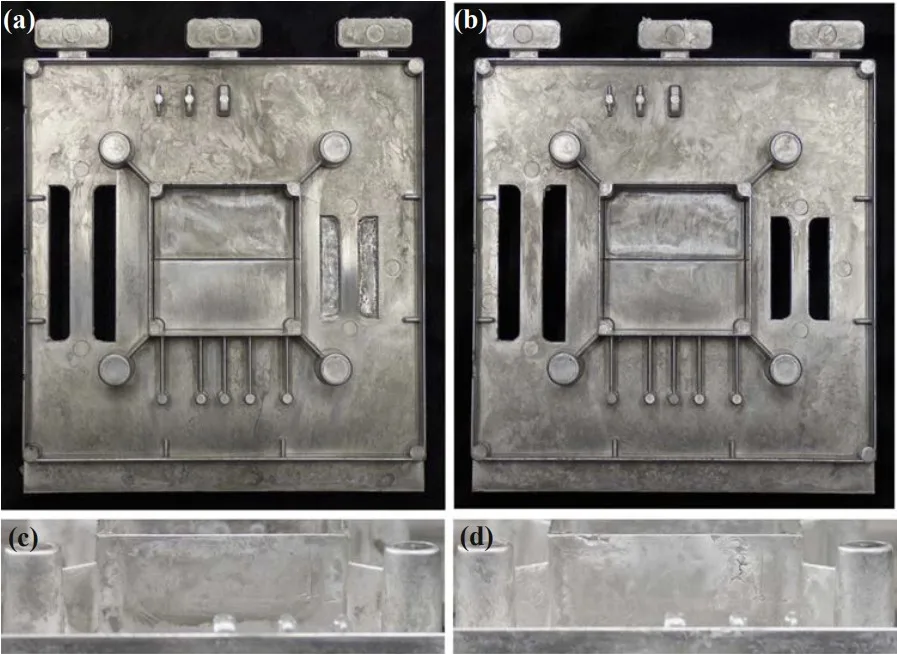
2. 연구 배경:
- 연구 주제의 사회적/학문적 맥락: 연비 향상 및 온실 가스 배출 감소에 대한 요구가 증가함에 따라 자동차 응용 분야에서 경량화의 필요성이 대두되고 있습니다. 고압 다이캐스팅 마그네슘 합금은 낮은 밀도와 높은 비강도로 인해 자동차 구조 부품에 유망한 후보 물질이며, 알루미늄, 강철 및 주철에 비해 상당한 무게 감소를 제공합니다. 구조용 합금은 충돌 상황 및 자가 피어싱 리벳팅과 같은 제조 공정에서 에너지 흡수를 위해 높은 강도와 연성을 가져야 합니다.
- 기존 연구의 한계: 아넬라스틱성이 마그네슘 합금 변형에 중요한 역할을 하지만, 탄성 및 소성에 비해 관심이 덜했습니다. 기존 연구는 아넬라스틱성에 대한 변형률 속도 및 알루미늄 함량의 영향에 대한 포괄적인 조사가 부족합니다.
- 연구의 필요성: 구조용 응용 분야를 위한 고압 다이캐스팅 마그네슘 합금의 기계적 성질을 향상시키기 위해서는 하중 하에서 이들 합금의 변형 거동에 대한 기본적인 이해가 필수적입니다. 본 연구는 이러한 합금의 변형 메커니즘을 조사하여 이러한 격차를 해소하는 것을 목표로 합니다.
3. 연구 목적 및 연구 질문:
- 연구 목적: 본 연구의 주요 목적은 상용 고압 다이캐스팅 마그네슘-알루미늄 기반 합금, 특히 Mg-4Al-0.3Mn (AM40), Mg-6Al-0.3Mn (AM60), Mg-9Al-1Zn (AZ91) 및 Mg-4Al-4RE (AE44)의 기계적 성질 및 변형 거동을 광범위한 변형률 속도 범위 10-6-10-1 s-1에서 조사하는 것입니다.
- 주요 연구 질문:
- 변형률 속도와 알루미늄 함량이 고압 다이캐스팅 마그네슘-알루미늄 기반 합금의 기계적 성질 및 변형 거동에 어떤 영향을 미치는가?
- 아넬라스틱성은 마그네슘 합금의 항복 강도 측정에 어떤 영향을 미치는가?
- 탄성, 아넬라스틱성 및 소성 변형이 전체 변형 거동에 개별적으로 기여하는 정도는 어느 정도이며, 변형률 속도 및 알루미늄 함량에 따라 어떻게 달라지는가?
- 변형 메커니즘(슬립 및 쌍정)은 응력-변형률 곡선의 여러 단계와 어떻게 관련될 수 있으며, 이러한 단계를 모델링하기 위한 구성 방정식을 개발할 수 있는가?
- 연구 가설:
- 아넬라스틱성은 마그네슘 합금의 항복 강도 측정에 모호성을 야기한다.
- 고압 다이캐스팅 마그네슘-알루미늄 합금의 변형률 속도 민감도는 α-마그네슘 기지 내 알루미늄 고용체 함량이 증가함에 따라 감소한다.
- 아넬라스틱성은 변형률 속도에 둔감하지만, 프리즘 슬립에 의한 소성은 변형률 속도에 민감하다.
4. 연구 방법:
- 연구 설계: 본 연구는 표준화된 항복 강도 측정 방법의 비판적 검토와 일련의 실험 연구를 포함하는 실험 설계를 채택했습니다. 이러한 연구는 다음 사항에 초점을 맞추었습니다.
- 다이캐스팅 마그네슘 합금의 항복 강도 측정.
- 변형률 속도 및 알루미늄 함량이 변형 거동에 미치는 영향.
- 다양한 변형률 속도에서 다이캐스팅 마그네슘-알루미늄 기반 합금의 아넬라스틱성.
- 상용 다이캐스팅 마그네슘-알루미늄 기반 합금의 변형 거동에 대한 구성 모델링.
- 데이터 수집 방법: 데이터는 다음을 통해 수집되었습니다.
- 광범위한 변형률 속도(10-6-10-1 s-1)에 걸친 단축 인장 시험.
- 아넬라스틱성을 특성화하기 위한 순환 인장 하중-제하 시험.
- 4가지 표준화된 항복 강도 측정 방법(방법 1, 방법 2, 방법 3, 방법 4)의 적용.
- 분석 방법: 수집된 데이터는 다음을 사용하여 분석되었습니다.
- 기계적 성질에 대한 통계적 및 질적 분석.
- 변형 단계 및 매개변수를 식별하기 위한 응력-변형률 곡선 분석.
- 변형 거동에 대한 예측 모델을 개발하기 위한 구성 모델링.
- 주사 전자 현미경(SEM), 에너지 분산형 X선 분광법(EDX) 및 전자 후방 산란 회절(EBSD)을 사용한 미세 구조 특성 분석.
- 연구 대상 및 범위: 연구는 상용 고압 다이캐스팅 마그네슘-알루미늄 기반 합금에 초점을 맞추었습니다.
- Mg-4Al-0.3Mn (AM40)
- Mg-6Al-0.3Mn (AM60)
- Mg-9Al-1Zn (AZ91)
- Mg-4Al-4RE (AE44)
- T5 시효 처리된 AE44
5. 주요 연구 결과:
- 주요 연구 결과:
- 항복 강도 측정: 아넬라스틱성은 표준화된 방법을 사용한 항복 강도 측정에서 불일치를 유발하는 것으로 나타났습니다. 고압 다이캐스팅 마그네슘 합금의 항복 강도 측정에서 보다 정확한 0.2% 잔류 소성 변형률을 달성하기 위해 0.45%의 더 높은 오프셋 변형률을 사용하는 실용적인 접근 방식이 제안되었습니다.
- 변형률 속도 민감도: 고용체 내 알루미늄 함량이 낮은 합금은 더 높은 변형률 속도 민감도를 나타내었으며, 이는 가공 경화 증가 및 인장/항복 강도비 증가로 나타났습니다. 알루미늄 고용체 함량이 증가함에 따른 변형률 속도 민감도 감소는 α-마그네슘 기지 내에서 동적 변형 시효 때문인 것으로 나타났습니다. 프리즘 및 피라미드 슬립의 높은 변형률 속도 민감도도 관찰되었습니다.
- 아넬라스틱성: 아넬라스틱성은 변형률 속도에 둔감한 반면, 프리즘 슬립에 의한 소성은 변형률 속도에 민감한 것으로 나타났습니다. 프리즘 슬립의 높은 변형률 속도 민감도는 변형률 속도에 따른 최대 아넬라스틱성의 변화를 야기했습니다. 용액 내 알루미늄 함량이 가장 높은 AZ91은 AM40 및 AM60에 비해 가장 큰 최대 아넬라스틱성을 나타냈습니다.
- 구성 모델링: 반경험적 모델이 개발되어 대부분의 합금에 대한 실험적 응력-변형률 곡선에 잘 부합하며, AZ91의 2-3단계 전환 영역에서 응력을 약간 과대평가했습니다.
- 통계적/질적 분석 결과:
- 방법 1에 의한 항복 강도 측정은 탄성 계수 E에 따라 달라지며, 응력이 증가함에 따라 45 GPa에서 38 GPa로 감소합니다.
- 시컨트 탄성 계수 Esec는 순환 시험에서 소성 변형률 및 루프 폭이 증가함에 따라 감소합니다.
- 아넬라스틱 변형률은 시험된 합금의 0.2% 영구 소성 변형률에서 약 0.25%입니다.
- 변형률 속도 민감도 m은 알루미늄 함량이 증가함에 따라 급격히 감소하여 3 wt.% Al 이상에서는 무시할 수 있게 됩니다.
- 3단계의 가공 경화율 Θ는 합금 조성에 따라 1~2 GPa로 다양합니다.
- 데이터 해석: 다이캐스팅 마그네슘 합금의 변형 거동은 복잡하며 탄성, 아넬라스틱성 및 소성의 세 가지 변형 영역으로 분리할 수 있습니다. 추가 분해를 통해 응력-변형률 곡선에서 탄성(1단계), 기저 슬립 및 쌍정(2단계), 프리즘 슬립(3단계) 및 피라미드 슬립(4단계)의 4단계를 확인할 수 있습니다.
- 그림 목록:
- Figure 2.14: An overview of the cyclic tension loading-unloading stress-strain curve of pure magnesium
- Figure 2.17: Anelastic strain (%) at 2% of plastic strain of pure magnesium, AZ91, Mg-0.8Zn and Mg-2.3Zn and Mg-1.5Gd as a function of grain size.
- Figure 2.18: The anelastic strain as a function of the applied tensile (solid lines) or compressive (dashed lines) plastic strain, for AZ91 in two different casting conditions: sand-cast (sc) and high-pressure die-cast (hpdc)
- Figure 2.19: Anelastic strain as a function of the tensile (solid lines) and compressive (dashed lines) plastic strain, for pure magnesium and various (a) zinc contents, (b) gadolinium contents and (c) aluminium contents.
- Figure 2.20: Stress-strain curves of HPDC AS21 with a best fit model of stress, σ as a function of strain, ε and strain rate, ε̇.
- Figure 2.21: Strain-rate sensitivity, m versus aluminium content (wt.%) for pure magnesium, and various ternary magnesium alloys.
- Figure 2.22: Strain-rate sensitivity, m as a function of strain rates covering from creep to dynamic strain rates, for pure magnesium and other ternary magnesium alloys.
- Figure 3.14: Room-temperature monotonic tensile curves of die-cast magnesium alloys AM40, AM60, AZ91, AE44 and T5-aged AE44 at strain rate, ε̇ of 10-4 s-1
- Figure 3.15: A comparison of monotonic and cyclic tensile curves of AM60 at strain rate 10-3 s-1
- Figure 1: Proof stress measurement methods: (a) Method 1, (b) Method 2 as specified in ASTM E8M-09 and (c) Method 3 and (d) Method 4 as stated in ISO 6892-1
- Figure 2: Proof stress values measurement by Method 1 for die-cast AE44, AM60 and AZ91 alloys
- Figure 3: Tensile curves of die-cast AE44, AM60, AZ91 and A380 showing respectively proof stress values measured by Method 2
- Figure 4: Cyclic stress-strain curve of AE44 showing proof stress measured by Method 3
- Figure 5: Cyclic stress-strain curve of A380 showing proof stress measurement by Method 3
- Figure 6: (a) Secant elastic modulus, Esec (GPa) and (b) anelastic strain (%) as a function of tensile permanent plastic strain (%)
- Figure 7: Proof stress measurement by Method 4 for AE44 where the alternative elastic modulus, Ealt is 33.3 GPa
- Figure 8: Inconsistency within Method 4 providing different alternative elastic moduli, Ealt, leading to a variation in proof stress values
- Figure 9: An overview of monotonic and cyclic stress-strain curves of die-cast (a) AE44, (b) AM60 and (c) AZ91
- Figure 10: Permanent plastic strain as a function of offset strain (plastic strain + anelastic strain) for die-cast magnesium alloys.
- Figure 11: Stress-strain curve ofAM60 showing a comparison of 0.2% and 0.5% offsets and the influence of errors in modulus determination
- Figure 1: True stress-strain curves of as-cast (a) AM40, (b) AM60, (c) AZ91, (d) AE44 and T5-aged (e) AE44 at different nominal strain rates, ε̇
- Figure 2: Effects of strain rate on (a) strength (MPa) and (b) elongation to fracture (%) of as-cast AM40, AM60, AZ91, AE44 and T5-aged AE44
- Figure 3: Variations of strain-rate sensitivity with (a) true strain, covering strain rates, ε̇ from 10-6-10-1 s-1 and (b) with strain rate at 3% strain.
- Figure 4: EBSD maps showing formation of different types of twins in as-cast AE44, AM60, and AZ91 specimens after tensile testing at different strain rates.
- Figure 5: Secondary electron images of fracture surfaces of (a, b) AM60, (c, d) AZ91 and (e, f) AE44 at different strain rates
- Figure 6: Backscattered electron images and EDX line profiles of the α-Mg matrix in (a) AM40, (b) AM60, (c) AZ91, (d) AE44, and (e) AE44-T5.
- Figure 7: A correlation of strain-rate sensitivity and Al solute concentration: (a) Inverse strain-rate sensitivity versus Al solute level in Mg phase and (b) strain-rate sensitivity as a function of sample diameter and thicknesses in die-cast AM60.
- Figure 1: An overview of cyclic stress-strain curve of die-cast AE44 at 10-4 s-1
- Figure 2: Monotonic flow curves of (a) AZ91 and (b) AE44 at strain rates 10-6-10-1 s-1
- Figure 3: Anelastic strain (defined in Fig. 1) as a function of strain, for as-cast (a) AM40, (b) AM60, (c) AZ91, (d) AE44 and (e) T5-aged AE44, at strain rate range 10-6-10-1 s-1
- Figure 4: EBSD maps of AE44 showing (a) twin-free microstructure in as-cast condition and the formation of different types of twins at (b,c) 10-6 s-1 and (d, e) 10-1 s-1 after cyclic testing to 3% strain.
- Figure 5: TEM observations of dislocations pile-ups at twin and grain boundaries in AE44 cyclic tested to 3% strain at (a) 10-6 s-1 and (c) 10-1 s-1
- Figure 6: A correlation between onsets of stages II and III with anelastic strain. (a) Monotonic tensile flow curve with the onsets of stage II marked by ‘O’ and stage III marked by ‘X’ and (b) stress as a function of anelastic strain at strain rate 10-4 s-1.
- Figure 7: Maximum anelastic strain as a function of strain rate, for all alloys tested.
- Figure 8: Offset strain (plastic strain + anelastic strain) as a function of permanent plastic strain for (a) AZ91 and (b) AE44 at strain rate range 10-6-10-1 s-1.
- Figure 1: Monotonic (dashed) and cyclic (solid) stress-strain behaviour of (a) AZ91 and (b) AE44 at different strain rates, ε̇ =10-6-10-2 s-1
- Figure 2: Applied stress as a function of Stage II strain, for as-cast (a) AM40, (b) AM60, (c) AZ91, (d) AE44 and (e) AE44-T5, at strain-rate range 10-6-10-1 s-1.
- Figure 3: Comparison of Weibull function and power law.
- Figure 4: Stress when εIII reaches 0.01 for as-cast AM40, AM60, AZ91, AE44 and T5-aged AE44 and 0.015 for AZ91 as a function of maximum anelastic stress.
- Figure 5: Average deformation behaviour of stage II for AZ91 and AE44, best fit using Eq. (3) (dashed) are compared with experiments (solid).
- Figure 6: Experimental flow curves (solid) of (a) AZ91 and (b) AE44 at strain rates 10-6-10-1 s-1 are compared with best fit linear lines (dashed) according to Eq. (4).
- Figure 7: The strain hardening rate, Θ in Stage III for present alloys at different strain rates.
- Figure 8: The prismatic yield stress, σy of present alloys at different strain rates.
- Figure 9: Experimental (solid) and best fit (dashed) stress-strain curve of stage IV for (a) AZ91 and (b) AE44.
- Figure 10: Comparison of Ludwik’s and Hollomon’s models with KIV and nIV of best fit of Eq. (13).
- Figure 11: Comparison of experimental (coloured) and modelled (black) stress-strain curves of (a) AM40, (b) AM60, (c) AZ91, (d) AE44, and (e) AE44-T5 at wide strain-rate range 10-6-10-1 s-1
- Figure 12: Enlarged stress-strain curve in the Stage II-III transition region for the present die-cast alloys.
- Figure 8.2: Comparison of (a) proof stress and (b) % elongation to fracture of magnesium and aluminium casting alloys.
- Figure 8.3: Fractions of (a) elastic and (b) anelastic + plastic for present die-cast magnesium alloys.
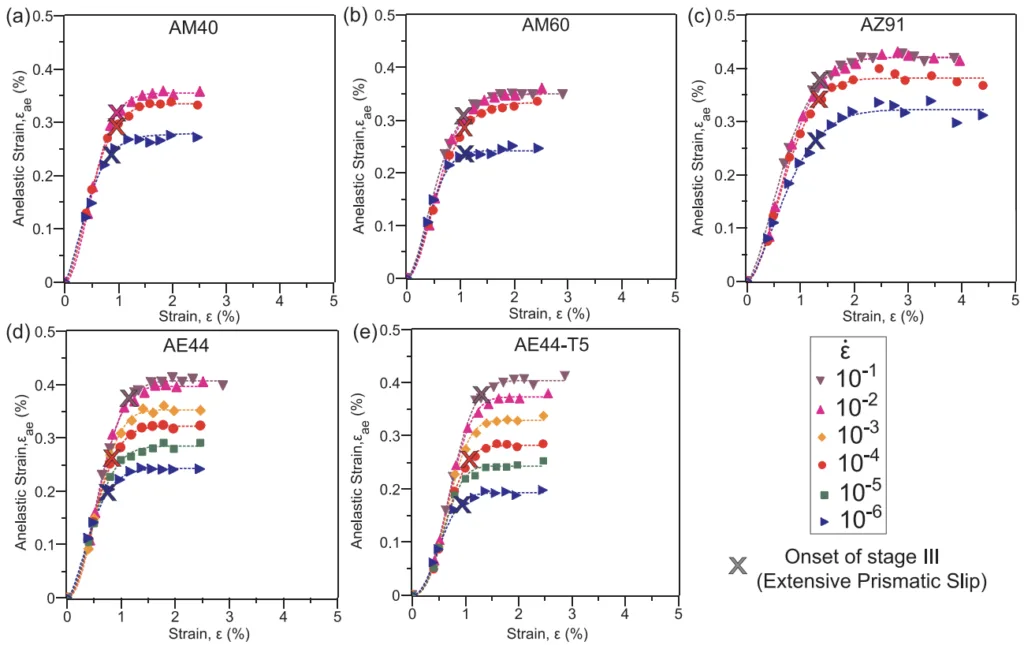
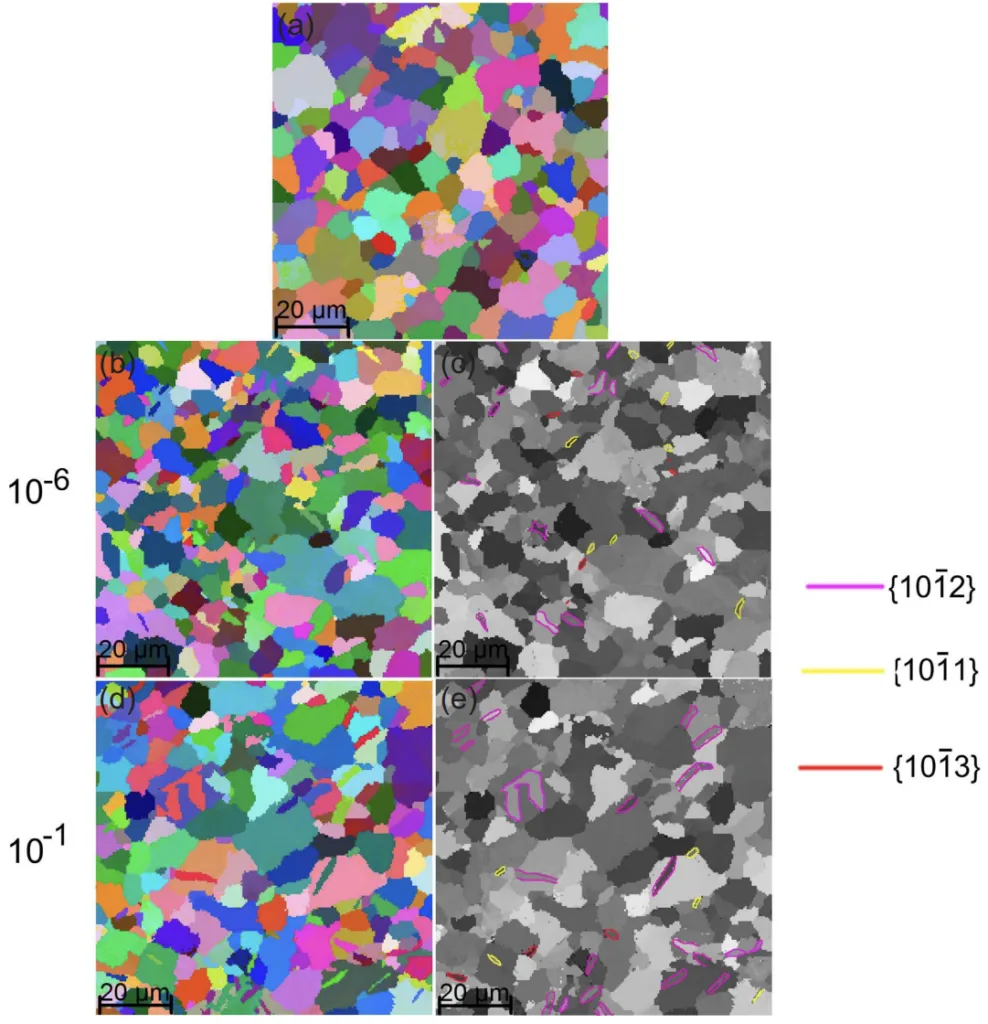
6. 결론 및 논의:
- 주요 결과 요약: 본 연구는 항복 강도 측정의 불일치, 변형률 속도 민감도, 아넬라스틱성을 해결하고 다이캐스팅 마그네슘-알루미늄 합금에 대한 구성 모델을 개발했습니다. 더 높은 오프셋 변형률 방법은 항복 강도 일관성을 개선했습니다. 변형률 속도 민감도는 α-마그네슘 기지 내 동적 변형 시효로 인해 알루미늄 고용체 함량이 증가함에 따라 감소했습니다. 아넬라스틱성은 변형률 속도에 둔감한 반면, 프리즘 슬립에 의한 소성은 변형률 속도에 민감했습니다. 4단계 구성 모델은 복잡한 변형 거동을 효과적으로 설명했습니다.
- 연구의 학문적 의의: 본 연구는 다이캐스팅 마그네슘 합금의 항복 강도 결정에 대한 보다 일관된 방법을 제공하여 재료 특성의 비교 가능성을 향상시킵니다. 항복 강도에 대한 기저 슬립 및 가역 쌍정의 영향을 명확히 하고, 이러한 합금의 변형 거동을 4개의 뚜렷한 단계에 걸쳐 포괄적으로 설명합니다. 이 연구는 변형률 속도 민감도를 조절하는 알루미늄 고용체의 역할과 아넬라스틱성을 결정하는 슬립 및 쌍정 메커니즘 간의 복잡한 상호 작용을 강조합니다.
- 실용적 의미: 제안된 일관된 항복 강도 결정 방법은 엔지니어링 응용 분야에서 마그네슘 합금 특성의 보다 신뢰할 수 있는 비교를 가능하게 합니다. 연구 결과는 마그네슘 합금, 특히 AE44가 더 높은 변형률 속도에서 향상된 에너지 흡수를 나타낼 수 있음을 시사하며, 이는 자동차 구조 응용 분야에서 충돌 안전성에 도움이 됩니다.
- 연구의 한계: 구성 모델은 AZ91의 2-3단계 전환 영역에서 응력을 약간 과대평가하여 고농도 합금에 대한 추가 개선의 필요성을 나타냅니다.
7. 향후 후속 연구:
- 후속 연구 방향: 향후 연구에서는 특히 준정적 변형률 속도 체제 외부에서 아넬라스틱성에 대한 변형률 속도 및 알루미늄 함량의 영향을 더 탐구해야 합니다. 구성 모델을 개선하고 향상된 구조용 합금을 개발하기 위해 변형 메커니즘, 특히 석출물 및 금속간 네트워크의 역할에 대한 추가 조사가 필요합니다.
- 추가 탐구가 필요한 영역:
- 더 높은 변형률 속도에서 아넬라스틱성에 대한 변형률 속도 및 알루미늄 함량의 영향.
- 석출물 및 금속간 네트워크가 아넬라스틱성 및 변형 거동에 영향을 미치는 정확한 메커니즘.
- 특히 AZ91과 같은 고알루미늄 함량 합금에서 2-3단계 전환 영역에서 정확도를 개선하기 위한 구성 모델의 개선.
- AE44-T5의 피로 특성 및 피로 수명에 대한 아넬라스틱 변형의 영향 조사.
8. 참고 문헌:
- [1] M.A. Meyers, Dynamic Behaviour of Materials, John Wiley & Sons, New York, 1994.
- [2] T.B. Abbott, M.A. Easton, R. Schmidt, Magnesium for crashworthy components, in: M. Byko (Ed.), Journal of The Minerals, Metals, and Materials Society, TMS Annual Meeting, California, 2003, pp. 227-230.
- [2] C.A. Newland, M.T. Murray, Strain rate dependent behaviour of magnesium-based alloys, in: Proceedings of the First Australasian Congress on Applied Mechanics, Institution of Engineers, Australia, 1996, pp. 73-76.
- [4] N. Stanford, M.R. Barnett, Effect of Al and Gd solutes on the strain rate sensitivity of magnesium alloys, Metall. Mater. Trans. A 41 (2010) 734-743.
- [5] T.K. Aune, D. Albright, H. Westengen, T.E. Johnsen, B. Anderson, Behavior of die cast magnesium alloys subject to rapid deformation, SAE Technical Paper, 2000 pp. 2000-01- 1116.
- [6] J.P. Weiler, J.T. Wood, Strain-rate effects of sand-cast and die-cast magnesium alloys under compressive loading, in: S.N. Mathaudhu, W.H. Sillekens, N.R. Neelameggham, N. Hort (Eds.), Magnesium Technology 2012, John Wiley & Sons, Florida, 2012, pp. 365-370.
- [7] A.V. Nagasekhar, M.A. Easton, C.H. Cáceres, Solute content and the grain microstructure of high pressure die cast magnesium–aluminium alloys, Adv. Eng. Mater. 11 (2009). 912- 919.
- [8] H.Q.Ang, S.M.Zhu, T.B.Abbott, Q.Dong, M.A.Easton, Strain-rate sensitivity of die-cast magnesium-aluminium based alloys
- [Accepted]. http://dx.doi.org/10.1016/j.msea.2017.05.093
- [9] H.Q. Ang, T.B. Abbott, S.M. Zhu, C.F. Gu, M.A. Easton, Proof stress measurement of diecast magnesium alloys, Mater. Des. 112 (2016) 402-409.
- [10] E. Karimi, A. Zarei-Hanzaki, M.H. Pishbin, H.R. Abedi, P. Changizian, Instantaneous strain rate sensitivity of wrought AZ31 magnesium alloy, Mater. Des. 49 (2013) 173-180.
- [11] W.S. Lee, T.H. Chen, Rate-dependent deformation and dislocation substructure of Al–Sc alloy, Scr. Mater. 54 (2006) 1463-1468.
- [12] T. Matsunaga, H. Somekawa, H. Hongo, M. Tabuchi, Deformation mechanism transition with strain rate in Mg–3Al–1Zn alloy at room temperature, Mater. Sci. Eng. A 647 (2015) 212-215.
- [13] R.B. Figueiredo, F. Poggiali, C. Silva, P.R. Cetlin, T.G. Langdon, The influence of grain size and strain rate on the mechanical behavior of pure magnesium, J. Mater. Sci. 51 (2016). 3012-3024.
- [14] X.Z. Lin, D.L. Chen, Strain hardening and strain-rate sensitivity of an extruded magnesium alloy, J. Mater. Eng. Perform. 17 (2008). 894-901.
- [15] L.P. Kubin, Y. Estrin, Dynamic strain ageing and the mechanical response of alloys, J. Phys. III 1 (1991) 929-943.
- [16] L. Jiang, J.J. Jonas, R. Mishra, Effect of dynamic strain aging on the appearance of the rare earth texture component in magnesium alloys, Mater. Sci. Eng. A 528 (2011) 6596-6605.
- [17] A. Van den Beukel, Theory of the effect of dynamic strain aging on mechanical properties, Phys. Stat. Sol. (a) 30 (1975) 197-206.
- [18] Z. Trojanová, P. Lukác, K.U. Kainer, V. Gärtnerová, Dynamic strain ageing during stress relaxation in selected magnesium alloys containing rare earth elements, Adv. Eng. Mater. 7 (2005) 1027-1032.
- [19] S.M. Zhu, J.F. Nie, M.A. Gibson, M.A. Easton, P. Bakke, Microstructure and creep behavior of high-pressure die-cast magnesium alloy AE44, Metall. Mater. Trans. A 43 (2012) 4137-4144.
- [20] S.M. Zhu, M.A. Easton, T.B. Abbott, M.A. Gibson, J.F. Nie, The influence of individual rare earth elements (La, Ce, or Nd) on creep resistance of die‐cast magnesium alloy AE44, Adv. Eng. Mater. 18 (2016) 932-937.
- [21] M.I. Khan, A.O. Mostafa, M. Aljarrah, E. Essadiqi, M. Medraj, Influence of cooling rate on microsegregation behavior of magnesium alloys, J. Mater. (2014) 1-18.
9. 저작권:
본 자료는 위 논문을 바탕으로 요약되었으며, 상업적 목적으로 무단 사용하는 것을 금지합니다.
Copyright © 2025 CASTMAN. All rights reserved.