본 논문 요약은 "Improving Electrical Conductivity of Commercially Pure Aluminium: The Synergistic Effect of AlB8 Master Alloy and Heat Treatment"제목으로 "Materials (MDPI)"에 발표된 논문을 기반으로 작성되었습니다.
1. 개요:
- 제목: 상업적으로 순수한 알루미늄의 전기 전도도 향상: AlB8 마스터 합금과 열처리 간의 시너지 효과 (Improving Electrical Conductivity of Commercially Pure Aluminium: The Synergistic Effect of AlB8 Master Alloy and Heat Treatment)
- 저자: 유수프 제이벡 (Yusuf Zeybek), 세밀레 카이쉬 (Cemile Kayış), 에게 아늘 딜러 (Ege Anıl Diler)
- 발행 연도: 2025년
- 발행 저널/학회: Materials (MDPI)
- 키워드: 상업적으로 순수한 알루미늄 (commercially pure aluminium), 전기 전도도 (electrical conductivity), 알루미늄-붕소 마스터 합금 (aluminium-boron master alloy), 결정립 성장 열처리 (grain-coarsening heat treatment), 전동기 효율 (efficiency of electrical motor)
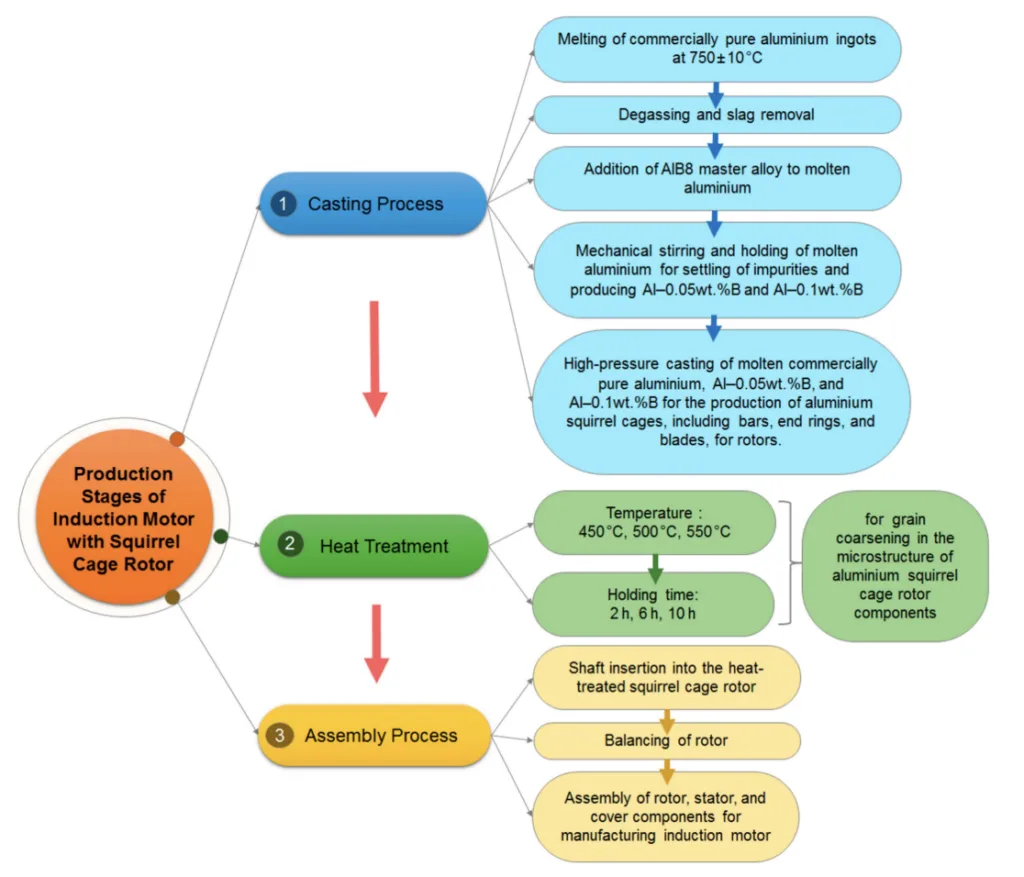
2. 연구 배경:
전 세계적으로 에너지 수요가 증가하고 온실가스 배출량 감축의 필요성이 대두됨에 따라 에너지 효율 향상이 중요한 과제로 떠올랐습니다. 산업 및 상업 분야에서 광범위하게 사용되는 전동기는 에너지 효율을 개선할 수 있는 중요한 영역입니다. 상업적으로 순수한 알루미늄 (CP-Al)은 낮은 밀도와 비용 효율성으로 인해 유도 전동기의 회전자 중 하나인 농형 회전자 제작에 자주 사용됩니다. 알루미늄은 상당한 전기 전도도를 가지고 있지만, 전동기 성능을 더욱 향상시키기 위해 전도도를 최적화할 필요가 있습니다.
알루미늄의 전기 전도도를 개선하기 위한 기존 방법들은 희토류 원소 사용을 포함하는 경우가 많습니다. 이러한 방법들은 효과적이지만 비용이 많이 듭니다. 알루미늄-붕소 (Al-B) 마스터 합금은 알루미늄 내 불순물 감소를 위한 보다 경제적인 대안을 제공합니다. 그러나 Al-B 마스터 합금의 적용은 결정립 미세화를 유도하여 결정립계 산란 증가로 인해 전기 전도도 향상 효과를 상쇄할 수 있습니다. 따라서 Al-B 마스터 합금의 불순물 제거 능력을 활용하면서도 결정립 미세화 효과를 완화하여 전기 전도도를 극대화하는 비용 효율적인 전략이 전기 모터 응용 분야의 CP-Al에 절실히 필요합니다.
3. 연구 목적 및 연구 질문:
본 연구는 상업적으로 순수한 알루미늄의 전기 전도도를 획기적으로 향상시키는 것을 목표로 합니다. 주요 목표는 알루미늄 미세 조직 내 불순물과 결정립계를 최소화하여 이 재료로 제작된 농형 회전자를 사용하는 전동기의 효율을 높이는 것입니다.
본 연구에서 다루는 핵심 연구 질문은 다음과 같습니다.
- AlB8 마스터 합금의 첨가가 상업적으로 순수한 알루미늄의 순도 수준과 전기 전도도에 어떤 영향을 미치는가?
- 결정립 성장 열처리가 상업적으로 순수한 알루미늄의 결정립 크기와 전기 전도도에 미치는 영향은 무엇인가?
- 상업적으로 순수한 알루미늄의 전기 전도도 향상에 AlB8 마스터 합금 첨가와 결정립 성장 열처리 사이에 시너지 효과가 존재하는가?
- 전기 전도도 향상이 전동기 효율 향상으로 어느 정도까지 이어지는가?
본 연구의 중심 가설은 AlB8 마스터 합금 첨가와 후속 결정립 성장 열처리의 조합 적용이 상업적으로 순수한 알루미늄의 전기 전도도를 시너지적으로 그리고 유의미하게 증가시켜 궁극적으로 전동기 효율의 측정 가능한 향상으로 이어진다는 것입니다.
4. 연구 방법론
본 연구는 통계적 실험 설계 및 반응 표면 방법론에 기반한 엄격한 연구 방법론을 채택하여 Box–Behnken 설계를 활용했습니다.
- 연구 설계: Box–Behnken 실험 설계를 구현하여 붕소 첨가 수준, 열처리 온도 및 유지 시간의 세 가지 요인이 상업적으로 순수한 알루미늄의 전기 전도도에 미치는 영향을 조사했습니다.
- 데이터 수집 방법: 전기 전도도 측정은 DIN EN 2004–1 및 ASTM E1004-17 표준에 따라 와전류 방법을 사용하는 SIGMASCOPE® SMP10 장비를 사용하여 수행했습니다. 미세 조직 특성 분석은 광학 현미경을 통해 수행했으며, 결정립 크기 분석은 ImageJ 소프트웨어를 사용하여 수행했습니다. 전동기 성능 평가는 KISTLER 전동기 테스트 장비를 사용하여 수행했습니다. 화학 조성 분석은 광학 방출 분광법을 통해 달성했습니다.
- 분석 방법: Design Expert Version 13 소프트웨어를 통해 지원되는 분산 분석 (ANOVA) 및 회귀 분석을 활용하여 요인 및 요인 간 상호 작용이 전기 전도도에 미치는 통계적 유의성을 평가했습니다. 변수와 반응 간의 관계를 시각화하기 위해 반응 표면 플롯과 등고선 플롯을 생성했습니다.
- 연구 대상 및 범위: 본 연구는 순도 99.7%의 상업적으로 순수한 알루미늄에 초점을 맞췄습니다. AlB8 마스터 합금을 도입하여 0.05 wt.% 및 0.1 wt.%의 붕소 첨가를 달성했습니다. 농형 회전자는 고압 다이캐스팅을 사용하여 제조했습니다. 결정립 성장 열처리는 450 °C, 500 °C 및 550 °C의 온도에서 2시간, 6시간 및 10시간의 유지 시간으로 수행했습니다.
5. 주요 연구 결과:
실험 결과 AlB8 마스터 합금 첨가와 결정립 성장 열처리의 시너지적 적용을 통해 상업적으로 순수한 알루미늄의 전기 전도도가 크게 향상되었음을 입증했습니다.
- 주요 연구 결과: 전기 전도도의 가장 큰 향상은 0.05 wt.% 붕소 첨가와 550 °C에서 10시간 동안의 결정립 성장 열처리 조합으로 달성되었습니다. 이 하이브리드 접근 방식은 초기 60.62% IACS에서 63.1% IACS로 전기 전도도를 증가시켰습니다. 이에 따라 이 방법을 사용하여 생산된 회전자로 제작된 전동기의 효율은 90.35%에서 91.53%로 증가했습니다. 분광 분석 결과 붕소 첨가가 불순물, 특히 티타늄 (Ti), 바나듐 (V) 및 지르코늄 (Zr)과 같은 전이 금속을 효과적으로 감소시킨 것으로 확인되었습니다. 미세 조직 분석 결과 열처리가 결정립 성장을 효과적으로 촉진하여 결정립계 밀도를 감소시킨 것으로 나타났습니다.
- 통계적/정성적 분석 결과: ANOVA 분석 결과 붕소 첨가, 열처리 온도, 유지 시간 및 이들의 2차 및 상호 작용 효과 (붕소 첨가와 유지 시간 간의 상호 작용 제외)가 전기 전도도에 유의미한 영향을 미치는 것으로 나타났습니다 (p < 0.05). 회귀 모델은 높은 결정 계수 (R² = 0.9859)를 나타내어 모델 적합성이 우수함을 나타냅니다.
- 데이터 해석: 전기 전도도 향상은 붕소 첨가에 의한 불순물 제거 효과와 결정립 성장 열처리를 통한 결정립계 산란 감소 효과의 조합으로 인한 것으로 해석됩니다. 시너지 효과는 우수한 전기적 성능을 달성하기 위해 재료 순도와 미세 조직 엔지니어링을 모두 최적화하는 것이 중요함을 강조합니다.
그림 목록:
- 그림 1. 농형 회전자, 열처리 적용 및 후속 유도 전동기 제조 단계의 생산 단계를 보여주는 다이어그램.
- 그림 2. 붕소 첨가를 통한 불순물 제거의 개략도.
- 그림 3. 고압 다이캐스팅 방법으로 상업적으로 순수한 알루미늄으로 제조된 농형 회전자 및 구성 요소.
- 그림 4. 농형 회전자 유도 전동기의 조립.
- 그림 5. 알루미늄 시편의 전기 전도도 측정.
- 그림 6. 유도 전동기의 성능 테스트.
- 그림 7. 중심점 (0,0,0)과 세 가지 요인의 12개 요인점을 갖는 Box-Behnken 설계 큐브.
- 그림 8. 비처리 및 열처리된 알루미늄 시편의 광학 현미경 사진.
- 그림 9. 비처리 알루미늄 시편의 결정립 크기 분석.
- 그림 10. 열처리된 Al-0.05B 시편의 결정립 크기 분석.
- 그림 11. 전기 전도도에 영향을 미치는 선형, 2차 및 상호 작용 항의 기여도.
- 그림 12. 예측된 전기 전도도 값과 실험적 전기 전도도 값 간의 상관 관계 그래프.
- 그림 13. 전기 전도도에 대한 (a) 붕소 첨가, (b) 열처리 온도 및 (c) 유지 시간의 주효과 플롯.
- 그림 14. (a) 2차원 등고선 플롯 및 (b) 3D 반응 표면 플롯은 붕소 첨가와 열처리 온도 간의 상호 작용 (A × T) 효과를 보여주고 (c) 2차원 등고선 플롯 및 (d) 3D 반응 표면 플롯은 열처리 온도와 유지 시간 간의 상호 작용 (T × H) 효과를 보여줍니다.
- 그림 15. 비처리 및 열처리된 CP-Al, Al-0.05B 및 Al-0.1B의 전동기 효율과 전기 전도도 간의 관계.
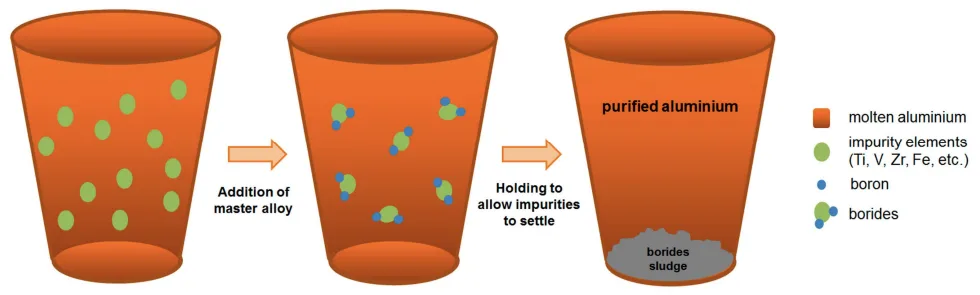
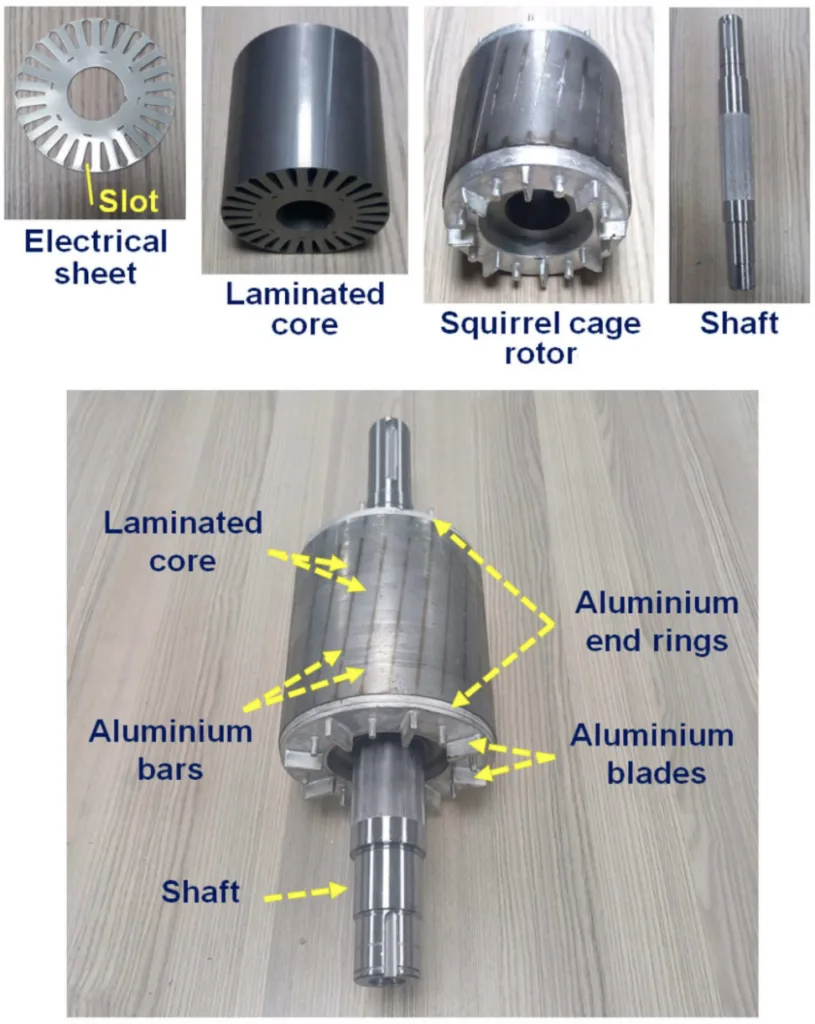
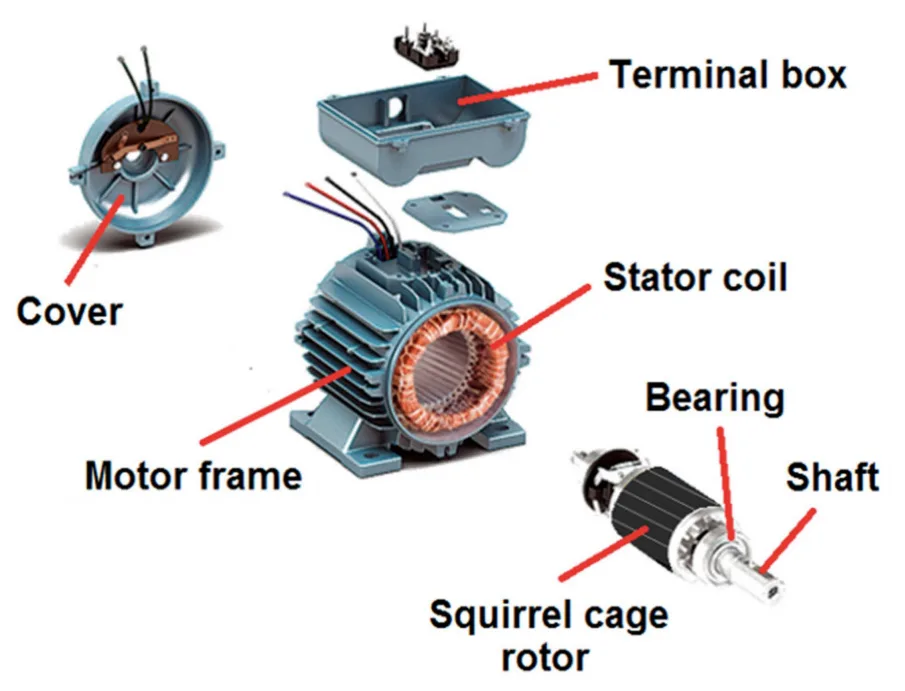
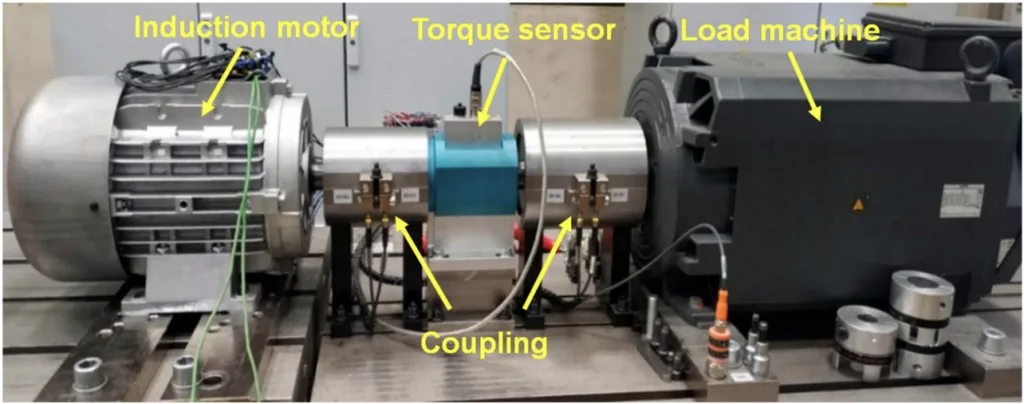
6. 결론 및 논의:
- 주요 결과 요약: 본 연구는 AlB8 마스터 합금 첨가와 결정립 성장 열처리를 결합한 하이브리드 접근 방식이 상업적으로 순수한 알루미늄의 전기 전도도를 향상시키는 효과적인 방법이며, 이는 전동기 효율의 현저한 개선으로 이어진다는 것을 성공적으로 입증했습니다.
- 연구의 학문적 의의: 본 연구는 금속 전도도를 최적화하기 위한 시너지 재료 가공 기술에 대한 기본적인 이해에 기여합니다. 또한 향상된 전기적 특성을 달성하는 데 있어 불순물 제어와 미세 조직 엔지니어링 간의 상호 작용을 강조합니다.
- 실용적 의미: 본 연구 결과는 상업적으로 순수한 알루미늄을 사용하는 전동기의 성능을 개선하기 위한 실용적이고 비용 효율적인 방법론을 제공합니다. 이 접근 방식은 다양한 산업 응용 분야에서 에너지 효율을 향상시키고 지속 가능한 제조 관행을 촉진하는 데 중요한 실용적 의미를 갖습니다.
- 연구의 한계: 본 연구는 전기 전도도 향상에 효과적으로 초점을 맞추었지만, 수정된 알루미늄의 기계적 특성에 우선적으로 초점을 맞추지는 않았습니다. 높은 전도도에도 불구하고 순수한 알루미늄의 적용 가능성은 높은 기계적 강도가 요구되는 시나리오에서는 제한적일 수 있습니다.
7. 향후 후속 연구:
- 후속 연구 방향: 향후 연구는 달성된 전기 전도도를 유지하거나 개선하면서 상업적으로 순수한 알루미늄의 기계적 특성을 더욱 향상시키는 데 초점을 맞춰야 합니다. 이는 다양한 합금 첨가 또는 대체 열처리 방안을 탐색하는 것을 포함할 수 있습니다.
- 추가 탐색이 필요한 영역: 열전도율 및 내식성과 같은 다른 중요한 재료 특성에 대한 이 하이브리드 접근 방식의 영향을 평가하기 위한 추가 연구가 필요합니다. 자동차 및 에너지 산업을 포함한 다양한 분야에서 이 향상된 상업적으로 순수한 알루미늄의 광범위한 산업 응용 분야를 탐색하는 것도 권장됩니다.
8. 참고 문헌:
- [1] De Almeida, A.T.; Ferreira, F.J.T.E.; Fong, J. Perspectives on electric motor market transformation for a net zero carbon economy. Energies 2023, 16, 1248.
- [2] Li, Z.; Hamidi, A.S.; Yan, Z.; Sattar, A.; Hazra, S.; Soulard, J.; Guest, C.; Ahmed, S.H.; Tailor, F. A circular economy approach for recycling electric motors in the end-of-life vehicles: A literature review. Resour. Conserv. Recycl. 2024, 205, 107582.
- [3] Mahale, M.D.; Patra, B.B. Efficient design, materials and specifications of electric motors used in electric vehicle challenges. In Energy Efficient Vehicles: Technologies and Challenges, 1st ed.; Singh, V.P., Kumar, A., Meena, C.S., Dwivedi, G., Eds.; CRC Press: Boca Raton, FL, USA, 2024; pp. 126–155.
- [4] Paul, K.; Sharma, D.; Konda, K.R.; Xie, T.; Höschler, K. Comprehensive review on cooling of permanent magnet synchronous motors and their qualitative assessment for aerospace applications. Energies 2023, 16, 7524.
- [5] Grassmann, R.M.; Shentu, C.; Hamoda, T.; Dewi, P.T.; Burgner-Kahrs, J. Open continuum robotics-one actuation module to create them all. Front. Robot. Al 2024, 11, 1272403.
- [6] Sun, B.; Zhang, Q.; Mao, H.; Li, Z. Validation of a statistical-dynamic framework for predicting energy consumption: A study on vehicle energy conservation equation. Energy Convers. Manag. 2024, 307, 118330.
- [7] Krzysztof, P.; Wnęk, H. Analysis of energy flow in hybrid and electric-drive vehicles. Energies 2024, 17, 1915.
- [8] Krishnamoorthy, S.; Panikkar, P.P.K. A comprehensive review of different electric motors for electric vehicles application. Int. J. Power Electron. Drive Syst. 2024, 15, 74–90.
- [9] Tong, W. Mechanical Design and Manufacturing of Electric Motors, 2nd ed.; CRC Press: Boca Raton, FL, USA, 2022; pp. 1–94.
- [10] Gobbi, M.; Sattar, A.; Palazzetti, R.; Mastinu, G. Traction motors for electric vehicles: Maximization of mechanical efficiency—A review. Appl. Energy 2024, 357, 122496.
- [11] Kumar, P.; Channi, H.K.; Singh, H. A Comprehensive Review of Electrical Motors for Electric Mobility. In Proceedings of 2024 IEEE International Conference for Women in Innovation, Technology & Entrepreneurship (ICWITE), Bangalore, India, 16–17 February 2024; pp. 130–135.
- [12] Manohar, V.J. Advantages and disadvantages of brushless DC motor. In Electrical Devices & Systems; Reddy, K.S., Ed.; CIIR Research Publications: Uttar Pradesh, India, 2023; pp. 20–22.
- [13] Mishra, A.; Agarwal, P.; Srivastava, S.P. A comprehensive analysis and implementation of vector control of permanent magnet synchronous motor. Int. J. Power Energy Convers. 2014, 5, 1–23.
- [14] Pindoriya, R.M.; Rajpurohit, B.S.; Kumar, R.; Srivastava, K.N. Comparative Analysis of Permanent Magnet Motors and Switched Reluctance Motors Capabilities for Electric and Hybrid Electric Vehicles. In Proceedings of 2018 IEEMA Engineer Infinite Conference (eTechNxT), New Delhi, India, 13–14 March 2018.
- [15] Jape, S.R.; Thosar, A. Comparison of electric motors for electric vehicle application. Int. J. Res. Eng. Technol. 2017, 6, 12–17.
- [16] Dimitrios, R.; Kaminaris, S.D.; Piromalis, D.D.; Vokas, G.; Arvanitis, K.G.; Karavas, C.S. Comparative review of motor technologies for electric vehicles powered by a hybrid energy storage system based on multi-criteria analysis. Energies 2023, 16, 2555.
- [17] Basma, B.; Cheghib, H.; Chrenko, D.; Delgado, M.T.; Hamoudi, Y.; Rodriguez, J.; Abdelrahem, M. Sliding mode control of an electric vehicle driven by a new powertrain technology based on a dual-star induction machine. World Electr. Veh. J. 2024, 15, 155.
- [18] Kurt, M.Ş. The use of induction motors in electric vehicles. In Induction Motors—Recent Advances, New Perspectives and Applications; El-Shahat, A., Ed.; IntechOpen: London, UK, 2023; pp. 1–10.
- [19] Thirugnanam, P. Advances, new perspective and applications in induction motors. In Induction Motors—Recent Advances, New Perspectives and Applications; El-Shahat, A., Ed.; IntechOpen: London, UK, 2023; pp. 1–15.
- [20] Su, H.; Wen, H.; Zheng, X.; Su, J. Development of a super high efficiency motor with boron aluminum alloy rotor. Procedia Eng. 2017, 174, 1221–1228.
- [21] Shadfari, H.; Izadfar, H.R. A new squirrel cage rotor structure to improve the dynamic performance of the single-phase induction motor. Int. J. Ind. Electron. Control. Optim. 2022, 5, 279–286.
- [22] Nandagopal, S.; Chokkalingam, L.N. Influence of squirrel cage induction rotor geometry in battery C-rating. Eng. Sci. Technol. 2023, 39, 101336.
- [23] Luthfiyah, H.; Qowiy, O.A.; Malakani, A.I.; Arthanto, D.H.; Setiawan, F.D.; Ramanel, T.A.; Putra, G.M.; Kamar, S.; Suryandi, A.A. An optimized stator and rotor design of squirrel cage induction motor for EMU train. J. Mechatron. Electr. Power Veh. Technol. 2023, 14, 35–46.
- [24] Wang, L.; Bao, X.; Di, C.; Zhou, Y. Influence on vibration and noise of squirrel-cage induction machine with double skewed rotor for different slot combinations. IEEE Trans. Magn. 2016, 52, 8104404.
- [25] Balcı, M.Ş.; Dalcalı, A. The effect of rotor slot number on the performance of asynchronous motors in light electric vehicle. Aintelia Sci. Notes 2023, 2, 40–46.
- [26] Dinh, B.M. Efficiency Improvement of Squirrel Cage Induction Motor by Rotor Slot Designs. In Proceedings of 13th International Conference on Industrial Engineering and Operations Management, Manila, Philippines, 7–9 March 2023; IEOM Society International: Manila, Philippines, 2023; pp. 615–623.
- [27] Konda, R.Y.; Ponnaganti, V.K.; Reddy, P.V.S.; Singh, R.R.; Mercorelli, P.; Gundabattini, E.; Solomon, D.G. Thermal analysis and cooling strategies of high-efficiency three-phase squirrel-cage induction motors—A review. Computation 2024, 12, 6.
- [28] Özsoy, M.; Kaplan, O.; Akar, M. FEM-Based Analysis of rotor cage material and slot geometry on double air gap axial flux induction motors. Ain Shams Eng. J. 2024, 15, 102393.
- [29] Marfoli, A.; Nardo, M.D.; Degano, M.; Gerada, C.; Jara, W. Squirrel cage induction motor: A design-based comparison between aluminium and copper cages. IEEE Open J. Ind. Appl. 2021, 2, 110–120.
- [30] Liu, Y.; Han, P.; Bazzi, A.M. A Comparison of Rotor Bar Material of Squirrel-Cage Induction Machines for Efficiency Enhancement Purposes. In Proceedings of 2015 17th European Conference on Power Electronics and Applications (EPE’15 ECCE-Europe), Geneva, Switzerland, 8–10 September 2015.
- [31] Ocak, C. A FEM-based comparative study of the effect of rotor bar designs on the performance of squirrel cage induction motors. Energies 2023, 16, 6047.
- [32] Agapiou, J.S. Development of manufacturing technology for a hybrid induction rotor. Manuf. Lett. 2023, 35, 277–288.
- [33] Mallard, V.; Parent, G.; Demian, C.; Brudny, J.-F.; Delamotte, A. Increasing the energy efficiency of induction machines by the use of grain-oriented magnetic materials and die casting copper squirrel cage in the rotor. IEEE Trans. Ind. Appl. 2019, 55, 1280–1289.
- [34] Peters, D.T.; Cowie, J.G.; Brush, E.F. Die casting copper motor rotors: Mold materials and processing for cost-effective manufacturing. In Energy Efficiency Improvements in Electronic Motors and Drives; Bertoldi, P., De Almeida, A.T., Falkner, H., Eds.; Springer: Berlin, Germany, 2000; pp. 39–51.
- [35] Peters, D.T.; Cowie, J.G.; Brush, E.F.; Midson, S.P. Die-cast copper motor rotors: Die materials and process considerations for economical copper rotor production. In Energy Efficiency in Motor Driven Systems; Parasiliti, F., Bertoldi, P., Eds.; Springer: Berlin, Germany, 2003; pp. 128–135.
- [36] Feng, Y.N.; Apsley, J.; Williamson, S.; Smith, A.C.; Ionel, D.M. Reduced losses in die-cast machines with insulated rotors. IEEE Trans. Ind. Appl. 2010, 46, 928–936.
- [37] Varghese, S.T.; Singh, B.; Rajagopal, K.R. Fault investigations on die-cast copper rotors. IEEE Trans. Ind. Appl. 2018, 54, 184–194.
- [38] Agapiou, J.S. Development on die-cast copper motor rotors—Casting considerations. Manuf. Lett. 2022, 33, 310–321.
- [39] Cowie, J.G.; Brender, D.T. Die-Cast Copper Rotors for Improved Motor Performance. In Proceedings of the Conference Record of the 2003 Annual Pulp and Paper Industry Technical Conference, Charleston, SC, USA, 16–20 June 2003; pp. 42–49.
- [40] Passarini, F.; Ciacci, L.; Nuss, P.; Manfredi, S. Material Flow Analysis of Aluminium, Copper, and Iron in the EU-28. EUR 29220 EN; Publications Office of the European Union: Luxembourg, 2018; ISBN 978-92-79-85744-7.
- [41] Lin, G.; Li, L.; Guo, Z.; Jia, X.; Wang, X.; Yuan, Z.; Zhang, G.; Zhan, Y.; Shan, Q.; Li, Z. Influence of cerium and yttrium addition on strength and electrical conductivity of pure aluminum alloys. J. Rare Earth. 2024, 42, 600–611.
- [42] Zhang, Y.; Wei, F.; Mao, J.; Niu, G. The difference of La and Ce as additives of electrical conductivity aluminum alloys. Mater. Charact. 2019, 158, 109963.
- [43] Medvedev, A.E.; Murashkin, M.Y.; Enikeev, N.A.; Valiev, R.Z.; Hodgson, P.D.; Lapovok, R. Enhancement of mechanical and electrical properties of Al-RE alloys by optimizing rare-earth concentration and thermo-mechanical treatment. J. Alloys Compd. 2018, 745, 696–704.
- [44] Murashkin, M.Y.; Sabirov, I.; Medvedev, A.E.; Enikeev, N.A.; Lefebvre, W.; Valiev, R.Z.; Sauvage, X. Mechanical and electrical properties of an ultrafine grained Al–8.5wt. % RE (RE=5.4wt.% Ce, 3.1wt.% La) alloy processed by severe plastic deformation. Mater. Des. 2016, 90, 433–442.
- [45] Cui, X.; Wu, Y.; Liu, X.; Zhao, Q.; Zhang, G. Effects of grain refinement and boron treatment on electrical conductivity and mechanical properties of AA1070 aluminum. Mater. Des. 2015, 86, 397–403.
- [46] Cui, X.; Wu, Y.; Cui, H.; Zhang, G.; Zhou, B.; Liu, X. The improvement of boron treatment efficiency and electrical conductivity of AA1070Al achieved by trace Ti assistant. J. Alloys Compd. 2018, 735, 62–67.
- [47] Karabay, S.; Uzman, I. Inoculation of transition elements by addition of AlB2 and AlB12 to decrease detrimental effect on the conductivity of 99.6% aluminium in CCL for manufacturing of conductor. J. Mater. Process. Technol. 2005, 160, 174–182.
- [48] Xu, X.; Feng, Y.; Fan, H.; Wang, Q.; Dong, G.; Li, G.; Zhang, Z.; Liu, Q.; Fan, X.; Ding, H. The grain refinement of 1070 alloy by different Al-Ti-B mater alloys and its influence on the electrical conductivity. Results Phys. 2019, 14, 102482.
- [49] Wang, T.; Chen, Z.; Fu, H.; Gao, L.; Li, T. Grain refinement mechanism of pure aluminum by inoculation with Al-B master alloys. Mater. Sci. Eng. A 2012, 549, 136–143.
- [50] Wang, T.; Chen, Z.; Fu, H.; Xu, J.; Fu, Y.; Li, T. Grain refining potency of Al-B master alloy on pure aluminum. Scr. Mater. 2011, 64, 1121–1124.
- [51] Dong, L.; Yang, F.; Yu, T.; Zhang, N.; Zhou, X.; Xie, Z.; Fang, F. Contribution of grain boundary to strength and electrical conductivity of annealed copper wires. J. Mater. Res. Technol. 2023, 26, 1459–1468.
- [52] Bakonyi, I. Accounting for the resistivity contribution of grain boundaries in metals: Critical analysis of reported experimental and theoretical data for Ni and Cu. Eur. Phys. J. Plus 2021, 136, 410.
- [53] Zheng, Q.; Zhang, B.; Chen, T.; Wu, J. Achieving superior grain refinement efficiency for Al-Si casting alloys through a novel Al-La-B grain refiner. J. Mater. Res. Technol. 2024, 30, 52–60.
- [54] Liu, G.; Ren, Y.; Ma, W.; Morita, K.; Lei, Y.; Zhan, S.; Lv, G.; Li, S.; Zeng, Y.; Li, R. Development process and future trends of chemical refining agents' influence on grain refinement in aluminum alloys. J. Mater. Res. Technol. 2024, 29, 242–257.
- [55] Sigworth, G.K. Grain refinement of Al-Si-Cu alloys by AlB2 and (Al,Ti)B2. Inter. Metalcast. 2024, 18, 2778–2782.
- [56] Birol, Y. Grain Refining Aluminium foundry alloys with commercial Al-B master alloys. Mater. Sci. Technol. 2014, 30, 277–282.
- [57] Khaliq, A.; Rhamdhani, M.A.; Brooks, G.A.; Grandfield, J. Analysis of boron treatment for V removal using AlB2 and AlB12 based master alloys. In Light Metals 2014; Grandfield, J., Ed.; Springer: Cham, Switzerland, 2014; pp. 963–968.
- [58] Yabiku, R.; Fialho, R.; Teran, L.; Santos, A.; Rangel, E.; Dutra, D. A Comparative Study between Copper and Aluminum Induction Squirrel Cage Constructions. In 2010 Record of Conference Papers Industry Applications Society 57th Annual Petroleum and Chemical Industry Conference (PCIC), San Antonio, TX, USA, 20–22 September 2010; pp. 1–9.
- [59] Czerwinski, F. Aluminum alloys for electrical engineering: A review. J. Mater. Sci. 2024, 59, 14847–14892.
- [60] Aboraia, M.; Elrab, H.G.G.; Abdalla, G. Joint addition of zirconium, titanium and chromium to commercial pure aluminium. J. P. Min. Eng. 2020, 22, 58–64.
- [61] Liu, J.; Wang, Y.; Cui, X. Study about the forming mechanism of boron in Al-1B alloy. Met. Mater. Int. 2024, in press.
- [62] Khaliq, A.; Rhamdhani, M.A.; Mitchell, J.B.; Davidson, C.J. Analysis of Transition Metal (V, Zr) Borides Formation in Aluminium Melt. In Proceedings of European Metallurgical Conference (EMC) 2011, Dusseldorf, Germany, 26–29 June 2011.
- [63] Khaliq, A.; Rhamdhani, M.A.; Brooks, G.A.; Grandfield, J. Performance Evaluation of AlB12 and AlB2 for the Boron Treatment of Molten Aluminium. In Proceedings of the 6th Annual High Temperature Processing Symposium 2014, Melbourne, Australia, 3–4 February 2014; pp. 75–78.
- [64] Barta, J.; Uzhegov, N.; Losak, P.; Ondrusek, C.; Mach, M.; Pyrhönen, J. Squirrel-cage rotor design and manufacturing for high-speed applications. IEEE Trans. Ind. Electron. 2019, 66, 6768–6778.
- [65] Gerada, D.; Mebarki, A.; Brown, N.L.; Bradley, K.J.; Gerada, C. Design aspects of high-speed high-power-density laminated-rotor induction machines. IEEE Trans. Ind. Electron. 2011, 58, 4039–4047.
- [66] Jo, S.H.; Lee, S.H. Changes in microstructure, mechanical properties, and electrical conductivity of severely drawn AA1070 wire with annealing. Korean J. Met. Mater. 2024, 62, 749–756.
- [67] Wang, Q.; Yang, P.; Zhang, B.; Fan, H.; Xu, X.; Li, W.; Fan, X.; Wang, J.; Ding, H. Microstructure and texture evolution of cold rolled 1070 Al alloy during the subsequent annealing treatment. Results Phys. 2019, 13, 102178.
- [68] DIN EN 2004-1; Aerospace Series; Test Methods for Aluminium and Aluminium Alloy Products; Part 1: Determination of Electrical Conductivity of Wrought Aluminium Alloys; German Institute for Standardisation: Berlin, Germany, 1993.
- [69] ASTM E1004-17; Test Method for Determining Electrical Conductivity Using the Electromagnetic (Eddy-Current) Method; ASTM International: West Conshohocken, PA, USA, 2017.
- [70] IACS. International Annealed Copper Standard (IACS); United States Department of Commerce: Washington, DC, USA, 1914.
- [71] TS EN 60034-2-1; Rotating Electrical Machines - Part 2-1: Standard Methods for Determining Losses and Efficiency from Tests (Excluding Machines for Traction Vehicles); Turkish Standards Institution: Ankara, Turkey, 2014.
- [72] ISO/IEC 17025:2017; General Requirements for the Competence of Testing and Calibration Laboratories; International Organization for Standardization and International Electrotechnical Commission: Geneva, Switzerland, 2017.
- [73] Olabinjo, O.O. Response surface techniques as an inevitable tool in optimization process. In Response Surface Methods—Theory, Applications and Optimization Techniques; Silva, V., Cardoso, J.S., Eds.; IntechOpen: London, UK, 2024; pp. 1–11.
- [74] Ferreira, S.L.C.; Bruns, R.E.; Ferreira, H.S.; Matos, G.D.; David, J.M.; Brandão, G.C.; da Silva, E.G.P.; Portugal, L.A.; dos Reis, P.S.; Souza, A.S.; et al. Box-Behnken design: An alternative for the optimization of analytical methods. Anal. Chim. Acta 2007, 597, 179–186.
- [75] Myers, R.H.; Montgomery, D.C.; Anderson-Cook, C.M. Response Surface Methodology: Process and Product Optimization Using Designed Experiments, 4th ed.; John Wiley & Sons: Hoboken, NJ, USA, 2016; pp. 1–825.
- [76] Iwundu, M.P.; Cosmos, J. The efficiency of seven-variable Box-Behnken experimental design with varying center runs on full and reduced model types. J. Math. Stat. 2022, 18, 196–207.
- [77] Elkady, E.F.; Fouad, M.A.; Mozayad, A.N. Application of Box-Behnken experimental design and response surface methodology for selecting the optimum RP-HPLC conditions for the simultaneous determination of methocarbamol, indomethacin and betamethasone in their pharmaceutical dosage form. BMC Chem. 2022, 6, 114.
- [78] Draper, N.R. Center points in second-order response surface designs. Technometrics 1982, 24, 127–133.
- [79] Hemavathi, M.; Varghese, E.; Shekhar, S.; Jaggi, S.; Bhowmik, A.; Sathianandan, T.V. Run order consideration for sequential third order rotatable designs. Commun. Stat. Simul. Comput. 2024, 53, 1068–1081.
- [80] Cooper, P.S.; Kearns, M.A. Removal of transition metal impurities in aluminium melts by boron additives. Mater. Sci. Forum 1996, 217-222, 141–146.
- [81] Khaliq, A. Thermodynamics and Kinetics of Transition Metal Borides Formation in Molten Aluminium. Ph.D. Thesis, Swinburne University of Technology, Melbourne, Australia, 2013.
- [82] Khaliq, A.; Rhamdhani, M.A.; Brooks, G.A.; Grandfield, J. Thermodynamic analysis of Ti, Zr, V and Cr impurities in aluminum melt. In Light Metals 2011; Lindsay, S.J., Ed.; Springer: Cham, Switzerland, 2011; pp. 751–756.
- [83] Gao, J.W.; Shu, D.; Wang, J.; Sun, B.D. Effect of boron on removal of iron from aluminium melts. Mater. Sci. Technol. 2009, 25, 1462–1466.
- [84] Imam, M.A. Phase Equilibria and Reaction Kinetics of Borides Based High-Temperature Thermoelectric Materials. Ph.D. Thesis, The University of Alabama, Tuscaloosa, AL, USA, 2018.
- [85] Gao, J.W.; Xie, M.X.; Xia, M.X. Effects of boron and lanthanum on the impurity iron in aluminium melts. Miner. Process. Extr. Metall.: Trans. Inst. Min. Metall. 2011, 120, 172–176.
- [86] Hatch, J.E. Aluminium: Properties and Physical Metallurgy, 1st ed.; ASM International: Metals Park, OH, USA, 1984; pp. 1–397.
- [87] Bishara, H.; Lee, S.; Brink, T.; Ghidelli, M.; Dehm, G. Understanding grain boundary electrical resistivity in Cu: The effect of boundary structure. ACS Nano 2021, 15, 15397–16946.
- [88] Subedi, K.N.; Kappagantula, K.; Kraft, F.; Nittala, A.; Drabold, D.A. Electrical conduction processes in aluminum: Defects and Phonons. Phys. Rev. B 2022, 105, 104114.
- [89] Samuel, E.; Tahiri, H.; Samuel, A.M.; Samuel, F.H. Heterogenous grain nucleation in Al-Si alloys: Types of nucleant inoculation. Metals 2024, 14, 271.
- [90] Park, S.B. Heterogeneous nucleation models to predict grain size in solidification. Prog. Mater. Sci. 2022, 123, 100822.
- [91] Burke, J.E.; Turnbull, D. Recrystallization and grain growth. Prog. Phys. Met. 1952, 3, 220–244.
- [92] Chen, Q.; Chen, R.; Su, J.; He, Q.; Tan, B.; Xu, C.; Huang, X.; Dai, Q.; Lu, J. The mechanisms of grain growth of Mg Alloys: A review. J. Magnes. Alloy. 2022, 10, 2384–2397.
- [93] Stange, H.; Brunken, S.; Greiner, D.; Heinemann, M.D.; Kaufmann, C.A.; Schmidt, S.S.; Bäcker, J.P.; Klaus, M.; Genzel, C.; Mainz, R. Diffusion-induced grain boundary migration as mechanism for grain growth and defect annihilation in chalcopyrite thin films. Acta Mater. 2016, 111, 377–384.
- [94] Tavakkoli, V.; Boltynjuk, E.; Scherer, T.; Mazilkin, A.; Ivanisenko, Y.; Ungar, T.; Kübel, C. Precipitate-mediated enhancement of mechanical and electrical properties in HPTE-processed Al-Mg-Si alloy. Mater. Sci. Eng. A 2024, 906, 146556.
- [95] Gaber, A.; Afify, N.; El-Halawany, S.M.; Mossad, A. Studies on Al-Mg solid solutions using electrical resistivity and microhardness measurements. EPJ Appl. Phys. 1999, 7, 103–109.
- [96] Hou, J.P.; Wang, Q.; Zhang, Z.J.; Tian, Y.Z.; Wu, X.M.; Yang, H.J.; Li, X.W.; Zhang, Z.F. Nano-scale precipitates: The key to high strength and high conductivity in al alloy wire. Mater. Des. 2017, 132, 148–157.
- [97] Vončina, M.; Paulin, I.; Medved, J.; Petrič, M. Predicting the quality of grain refiners from electrical resistance measurements of aluminum. Metals 2023, 13, 717.
- [98] Murashkin, M.; Medvedev, A.; Kazykhanov, V.; Krokhin, A.; Raab, G.; Enikeev, N.; Valiev, R.Z. Enhanced mechanical properties and electrical conductivity in ultrafine-grained Al 6101 alloy processed via ECAP-conform. Metals 2015, 5, 2148–2164.
- [99] Yang, Z.; Mallow, S.; Banhart, J.; Kessler, O. Probing precipitation in aluminium alloys during linear cooling via in-situ differential scanning calorimetry and electrical resistivity measurement. Thermochim. Acta 2024, 739, 179815.
- [100] Eivani, A.; Ahmed, H.; Zhou, J.; Duszczyk, J. Correlation between electrical resistivity, particle dissolution, precipitation of dispersoids, and recrystallization behavior of AA7020 aluminum alloy. Metall. Mater. Trans. A 2009, 40, 2435–2446.
- [101] Wang, M.; Wu, J.; Yang, S.; Knezevic, M.; Huang, Z.; Zhao, Y.; Liu, T.; Shen, B.; Wang, J. Processing of an as-cast Al-7.5 wt%Y eutectic alloy by rolling and annealing to improve the tradeoff between strength and electrical conductivity. Mater. Sci. Eng. A 2024, 890, 145950.
- [102] Vandersluis, E.; Ravindran, C.; Bamberger, M. Mechanisms affecting hardness and electrical conductivity in artificially-aged B319 aluminum alloy. J. Alloys Compd. 2021, 867, 159121.
- [103] Li, Y.F.; Qin, J.; Yu, J.M.; Liu, Y.; Li, Z.; Nagaumi, H.; Zhang, B. A novel approach to improve conductivity of 1XXX Al alloy by in situ synthesis of Al-X-B grain refiner. Metall. Mater. Trans. A 2024, 55, 1707–1712.
- [104] Belov, N. Multicomponent Phase diagrams: Applications for Commercial Aluminum Alloys; Elsevier: Amsterdam, The Netherlands, 2005; pp. 1-413.
- [105] Sauvage, X.; Bobruk, E.V.; Murashkin, Y.M.; Nasedkina, Y.; Enikeev, N.A.; Valiev, R.Z. Optimization of electrical conductivity and strength combination by structure design at the nanoscale in Al-Mg-Si alloys. Acta Mater. 2015, 98, 355–366.
- [106] De Souza, D.F.; Salotti, F.A.M.; Sauer, I.L.; Tatizawa, H.; De Almeida, A.T.; Kanashiro, A.G. A performance evaluation of three-phase induction electric motors between 1945 and 2020. Energies 2022, 15, 2002.
9. 저작권:
본 자료는 유수프 제이벡, 세밀레 카이쉬, 에게 아늘 딜러의 논문: "[논문 제목]" (Improving Electrical Conductivity of Commercially Pure Aluminium: The Synergistic Effect of AlB8 Master Alloy and Heat Treatment)을 기반으로 합니다.
논문 출처: https://doi.org/10.3390/ma18020364
본 자료는 상기 논문을 기반으로 요약되었으며, 상업적 목적으로 무단 사용하는 것을 금지합니다.
Copyright © 2025 CASTMAN. All rights reserved.