1. 개요:
- 제목: Design of gravity assisted heat exchanger and its application on enhanced waste heat recuperation utilizing organic Rankine and LNG system
- 저자: Rizvi Arefin Rinik, Naimul Islam, M. Monjurul Ehsan, Yasin Khan
- 발행 연도: 2024
- 발행 학술지/학회: International Journal of Thermofluids
- Keywords: Waste Heat, Gravity heat exchanger, Organic Rankine, Combined cycle, Parametric optimization
2. 연구 배경:
세계 에너지 수요는 2050년까지 33% 증가할 것으로 예상되며, 화석연료 고갈과 지속가능성 문제 해결을 위해 폐열회수 기술의 중요성이 커지고 있다. 기존 폐열회수 기술은 초기 투자비용이 높고, 폐열원의 온도 제약, 오염된 배기가스 처리의 어려움 등의 한계를 가지고 있다. 본 연구는 중저온 폐열을 효율적으로 회수하기 위해 중력을 이용한 새로운 열교환기 시스템을 제안하고, 유기랭킨사이클(ORC)과 액화천연가스(LNG) 사이클을 결합하여 에너지 회수 효율을 극대화하고자 한다.
3. 연구 목적 및 연구 질문:
- 연구 목적: 중력 보조 열교환기 설계 및 ORC-LNG 결합 시스템을 통한 폐열 회수 효율 향상
- 핵심 연구 질문:
- 중력 보조 열교환기를 이용한 폐열 회수 효율은 어느 정도인가?
- ORC 및 LNG 사이클의 최적 운전 조건은 무엇인가?
- 결합 시스템의 에너지 효율 및 경제성은 어떠한가?
- 연구 가설: 중력 보조 열교환기와 ORC-LNG 결합 시스템은 기존 기술 대비 향상된 폐열 회수 효율과 경제성을 제공할 것이다.
4. 연구 방법론:
- 연구 설계: 중력 보조 열교환기, ORC, LNG 사이클을 포함하는 통합 에너지 회수 시스템 설계 및 성능 평가. 수치적 최적화 모델 개발 및 경제성 분석 수행.
- 데이터 수집 방법: 열전달 해석, 에너지 효율 시험, 에너지 분석 및 경제성 분석을 통한 데이터 수집. 실험 데이터와의 검증을 통해 모델의 정확성을 확인.
- 분석 방법: 열전달 해석, 에너지 효율 및 에너지 분석, 최적화 모델링 (수학적 모델 및 알고리즘), 경제성 분석
- 연구 대상 및 범위: 섬유 산업의 열처리 공정에서 발생하는 폐열을 대상으로 연구 수행. 중력 보조 열교환기의 설계 매개변수 최적화 및 ORC, LNG 사이클 운전 조건 최적화
5. 주요 연구 결과:
- 핵심 발견사항:
- 중력 보조 열교환기는 최적 온도 275-280°C, 공기 유량 35 kg/s에서 약 52.3%의 효율을 보였다.
- 펜테인을 작동유체로 사용하는 ORC 사이클은 응축기 압력 0.21 MPa에서 최대 280 kW의 출력과 36.8%의 효율을 달성했다.
- 응축기 압력 증가에 따라 ORC 터빈 출력은 220 kW에서 240 kW로, LNG 터빈 출력은 25 kW에서 40 kW로 증가했다.
- 핀치 온도가 7°C 증가하면 시스템의 에너지 효율은 4.94% 감소한다.
- 경제성 분석 결과, 설계된 중력 보조 열교환기는 기존 열교환기 대비 경제적으로 타당성이 있는 것으로 나타났다.
- 통계적/정성적 분석 결과: 표 8-12, 그림 5-14, 그림 16-17, 그림 19. (자세한 수치 및 그래프는 논문 참조)
- 데이터 해석: 다양한 작동 유체(펜테인, 노벡-649, 이소부탄, R245fa, R123)에 대한 ORC 사이클의 최적 운전 조건을 분석하고, 중력 보조 열교환기의 설계 변수(튜브 개수, 핀치 온도, 응축기 압력 등)가 시스템 성능에 미치는 영향을 평가.
- Figure Name:
- 그림 1 (a): setting machine에서 중력 열파이프 열교환기 다이어그램, (b): 중력 열파이프 작동 메커니즘 (5)
- 그림 2: 설계된 중력 파이프 열교환기 개략도 (5)
- 그림 3: 유기 랭킨 및 LNG 시스템을 활용한 폐열 회수를 위한 제안된 복합 사이클의 개략도 및 T-s 다이어그램 (6)
- 그림 4: 제안된 통합 시스템의 수학적 모델링 프레임워크 (8)
- 그림 5 (a): 핀튜브 길이에 따른 공기 측 압력 강하, (b): 배기가스 유량에 따른 응축기 출구 온도 (9)
- 그림 6 (a): 열전달 계수와 고온 공기 유량의 관계, (b): 고온 공기 입구에서 튜브 번들 수에 따른 압력 강하 (10)
- 그림 7 (a): 다른 고온 공기 유량에서 열전달 속도, (b): 증발기 입구에서 다른 질량 유량에서 열교환기 효율 (11)
- 그림 8 (a): 터빈 입구 압력이 시스템 질량 유량과 순출력에 미치는 영향, (b): 다른 작동 유체에 대한 ORC 터빈 입구 압력과 순출력의 관계 (11)
- 그림 9 (a): ORC 터빈 입구 온도가 사이클 효율에 미치는 영향, (b): ORC 터빈 입구 온도가 순출력에 미치는 영향 (11)
- 그림 10: ORC 응축기 압력이 순출력과 질량 유량에 미치는 영향 (12)
- 그림 11: ORC 응축기 압력이 총 에너지 효율과 순출력에 미치는 영향 (13)
- 그림 12: ORC 작동 유체가 ORC 열효율에 미치는 영향 (13)
- 그림 13 (a): 열원 온도가 에너지 효율에 미치는 영향, (b): 열원 온도가 제2법칙 효율 및 사이클 순출력의 함수로서의 열원 온도 (14)
- 그림 14 (a): 핀치 온도가 순출력과 에너지 효율에 미치는 영향, (b): 핀치 온도가 압력 강하에 미치는 영향 (14)
- 그림 15: 다른 열원 온도에서 구성 요소별 에너지 파괴 (15)
- 그림 16: ORC 터빈 입구 압력에 따른 에너지 효율 및 에너지 파괴 (15)
- 그림 17: 다른 응축기 압력에서 구성 요소별 에너지 파괴 (15)
- 그림 18: 기존 HE를 가진 제안된 GPHE 시스템의 경제 분석 모델링 프레임워크 (16)
- 그림 19 (a): 열전달 면적을 기준으로 한 비용 연구, (b): 구성 요소별 고급 분석 (17)
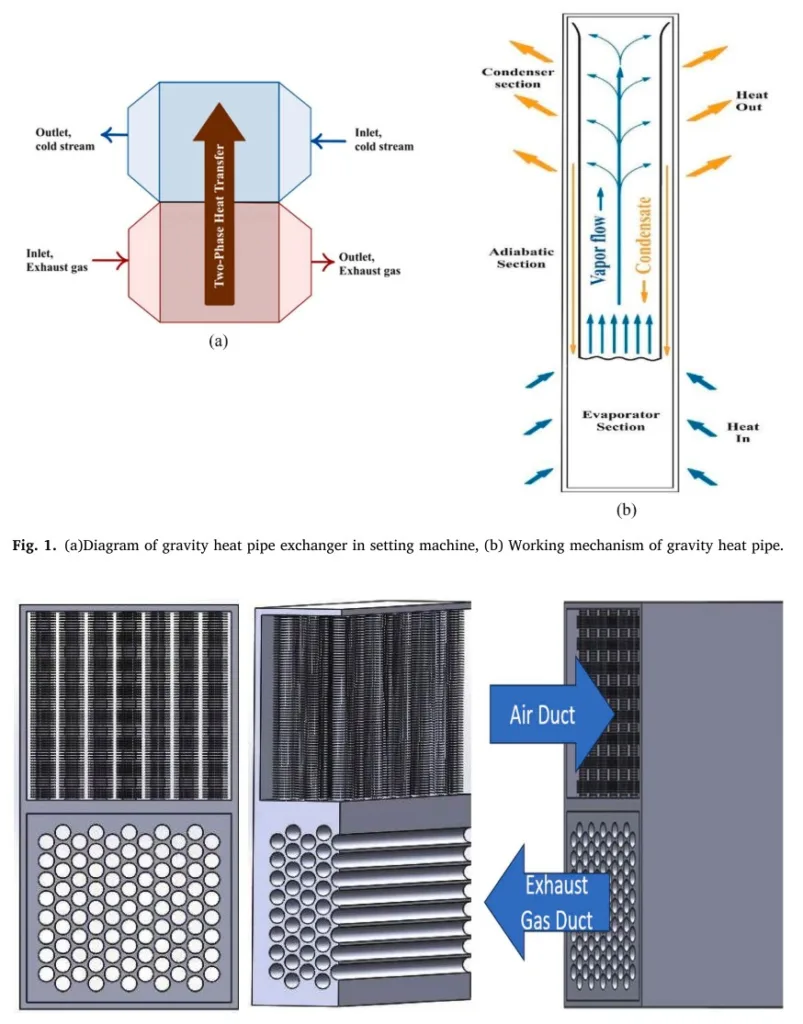
6. 결론 및 논의:
본 연구는 중력 보조 열교환기와 ORC-LNG 결합 시스템을 이용한 폐열 회수 시스템의 설계 및 성능 평가를 수행했다. 연구 결과, 제안된 시스템은 기존 기술 대비 높은 폐열 회수 효율과 경제성을 확보할 수 있음을 보여주었다. 특히, 중력 보조 열교환기는 오염된 배기가스에서도 효과적인 열교환이 가능하며, ORC와 LNG 사이클의 최적 운전 조건을 통해 시스템 성능을 극대화할 수 있었다. 본 연구는 폐열 회수 기술 발전에 기여하며, 산업 현장의 에너지 효율 향상과 환경 개선에 중요한 시사점을 제공한다. 그러나, 실제 산업 현장 적용을 위한 추가 연구가 필요하다. 특히, 장기간 운전 안정성 및 내구성 검증, 다양한 폐열원에 대한 적용 가능성 확대, 시스템 통합 및 제어 기술 개발 등에 대한 후속 연구가 필요하다.
7. 향후 후속 연구:
- 후속 연구 방향: 실제 산업 현장 적용을 위한 시험 및 검증, 다양한 폐열원 및 작동 유체에 대한 추가 연구, 장기 운전 안정성 및 내구성 평가, 시스템 제어 및 최적화 기술 개발, 경제성 분석 고도화, 다양한 국가 및 산업에 대한 적용 가능성 평가
- 추가 탐구가 필요한 영역: 다양한 폐열원 조건(온도, 유량, 오염도 등)에 대한 시스템 성능 분석, 다양한 작동 유체의 비교 및 최적화, 시스템 안정성 및 제어 기술 개발, 장기 운전에 따른 마모 및 부식 등 내구성 평가, 시스템의 경제성 및 환경적 영향 평가, 다른 산업 분야(발전, 선박 등)에 대한 적용 가능성 연구
8. 참고문헌 요약:
본 요약문에는 70개 이상의 참고문헌이 포함되어 있으며, 각 참고문헌은 폐열 회수 기술, 유기랭킨사이클, 액화천연가스 사이클, 중력 보조 열교환기, 에너지 효율 및 경제성 분석 등과 관련된 선행 연구들을 다루고 있다.
- [1] M.A. Islam, H.S. Che, M. Hasanuzzaman, N.A. Rahim, Energy demand forecasting. Energy for Sustainable Development, Elsevier, 2020, pp. 105–123, https://doi.org/ 10.1016/B978-0-12-814645-3.00005-5.
- [2] D.M. Arias, O. García-Valladares, G. Besagni, C.N. Markides, A vision of renewable thermal technologies for drying, biofuels production and industrial waste, gas or water recovery, Appl. Therm. Eng. 223 (2023) 120022, https://doi.org/10.1016/j. applthermaleng.2023.120022.
- [3] S.S. Siwal, et al., Recovery processes of sustainable energy using different biomass and wastes, Renew. Sustain. Energy Rev. 150 (2021) 111483, https://doi.org/ 10.1016/j.rser.2021.111483.
- [4] S. Nallathambi, K. Ramasamy, Prediction of electricity consumption based on DT and RF: an application on USA country power consumption, in: 2017 IEEE International Conference on Electrical, Instrumentation and Communication Engineering (ICEICE), IEEE, 2017, pp. 1–7, https://doi.org/10.1109/ ICEICE.2017.8191939.
- [5] D. Pashchenko, Natural gas reforming in thermochemical waste-heat recuperation systems: a review, Energy 251 (Jul. 2022) 123854, https://doi.org/10.1016/j. energy.2022.123854.
- [6] Y. Zhao, et al., A comparative study of energy consumption and efficiency of Japanese and Chinese manufacturing industry, Energy Policy 70 (2014) 45–56, https://doi.org/10.1016/j.enpol.2014.02.034.
- [7] B. Liu, M. Lu, B. Shui, Y. Sun, W. Wei, Thermal-hydraulic performance analysis of printed circuit heat exchanger precooler in the Brayton cycle for supercritical CO2 waste heat recovery, Appl. Energy 305 (2022) 117923, https://doi.org/10.1016/j. apenergy.2021.117923.
- [8] S.M. Hosseinnia, M. Sorin, A systematic pinch approach to integrate stratified thermal energy storage in buildings, Energy Build. 232 (2021) 110663, https://doi. org/10.1016/j.enbuild.2020.110663.
- [9] Z. Zhang, L. Zhang, Y. Yuan, Numerical simulation of turbulent natural convection heat transfer in an MW-class offshore wind turbine nacelle based on a multi-feature acquisition meshing technique, Sustain. Energy Technol. Assess. 57 (2023) 103249, https://doi.org/10.1016/j.seta.2023.103249.
- [10] D. Brough, J. Ramos, B. Delpech, H. Jouhara, Development and validation of a TRNSYS type to simulate heat pipe heat exchangers in transient applications of waste heat recovery, Int. J. Thermofluids 9 (2021) 100056, https://doi.org/ 10.1016/j.ijft.2020.100056.
- [11] E. Tian, Y.-L. He, W.-Q. Tao, Research on a new type waste heat recovery gravity heat pipe exchanger, Appl. Energy 188 (2017) 586–594, https://doi.org/10.1016/ j.apenergy.2016.12.029.
- [12] L.Y. Zhang, et al., Experimental investigation and economic analysis of gravity heat pipe exchanger applied in communication base station, Appl. Energy 194 (2017) 499–507, https://doi.org/10.1016/j.apenergy.2016.06.023.
- [13] X. Liu, X. Han, Z. Wang, G. Hao, Z. Zhang, Y. Chen, Application of an anti-gravity oscillating heat pipe on enhancement of waste heat recovery, Energy Convers. Manage 205 (2020) 433–444, https://doi.org/10.1016/j.enconman.2019.112404.
- [14] Z. Guo, J. Yang, Z. Tan, X. Tian, Q. Wang, Numerical study on gravity-driven granular flow around tube out-wall: Effect of tube inclination on the heat transfer, Int. J. Heat. Mass Transf. 174 (2021) 121296, https://doi.org/10.1016/j. ijheatmasstransfer.2021.121296.
- [15] H. Jouhara, N. Nieto, B. Egilegor, J. Zuazua, E. Gonz´alez, I. Yebra, A. Igesias, B. Delpech, S. Almahmoud, D. Brough, J. Malinauskaite, A. Vlasopoulos, M. Hill, B. Axcell, Waste heat recovery solution based on a heat pipe heat exchanger for the aluminium die casting industry, Energy 266 (2023), https://doi.org/10.1016/j. energy.2022.126459.
- [16] M. Imran, R. Pili, M. Usman, F. Haglind, Dynamic modeling and control strategies of organic Rankine cycle systems: methods and challenges, Appl. Energy 276 (2020) 115537, https://doi.org/10.1016/j.apenergy.2020.115537.
- [17] P.R. Gupta, A.K. Tiwari, Z. Said, Solar organic Rankine cycle and its polygeneration applications – a review, Sustain. Energy Technol. Assess. 49 (2022) 101732, https://doi.org/10.1016/j.seta.2021.101732.
- [18] R. Loni, et al., A critical review of power generation using geothermal-driven organic Rankine cycle, Therm. Sci. Eng. Progr. 25 (2021) 101028, https://doi.org/ 10.1016/j.tsep.2021.101028.
- [19] C. Wieland, et al., Innovations for organic Rankine cycle power systems: current trends and future perspectives, Appl. Therm. Eng. 225 (2023) 120201, https://doi. org/10.1016/j.applthermaleng.2023.120201.
- [20] S. Hu, J. Li, F. Yang, Z. Yang, Y. Duan, Multi-objective optimization of organic Rankine cycle using hydrofluorolefins (HFOs) based on different target preferences, Energy 203 (2020) 117848, https://doi.org/10.1016/j. energy.2020.117848.
- [21] X. Li, et al., Organic Rankine cycle systems for engine waste-heat recovery: Heat exchanger design in space-constrained applications, Energy Convers. Manage 199 (2019) 111968, https://doi.org/10.1016/j.enconman.2019.111968.
- [22] M.A. Chatzopoulou, S. Lecompte, M. De Paepe, C.N. Markides, Off-design optimisation of organic Rankine cycle (ORC) engines with different heat exchangers and volumetric expanders in waste heat recovery applications, Appl. Energy 253 (2019) 113442, https://doi.org/10.1016/j.apenergy.2019.113442.
- [23] Z. Zheng, J. Cao, W. Wu, M.K.H. Leung, Parallel and in-series arrangements of zeotropic dual-pressure Organic Rankine Cycle (ORC) for low-grade waste heat recovery, Energy Rep. 8 (2022) 2630–2645, https://doi.org/10.1016/j. egyr.2022.01.057.
- [24] J. Song, X. Li, K. Wang, C.N. Markides, Parametric optimisation of a combined supercritical CO2 (S-CO2) cycle and organic Rankine cycle (ORC) system for internal combustion engine (ICE) waste-heat recovery, Energy Convers. Manage 218 (Aug. 2020) 112999, https://doi.org/10.1016/j.enconman.2020.112999.
- [25] Ł. Witanowski, P. Klonowicz, P. Lampart, T. Suchocki, Ł. Jędrzejewski, D. Zaniewski, P. Klimaszewski, Optimization of an axial turbine for a small scale ORC waste heat recovery system, Energy 205 (2020) 118059, https://doi.org/ 10.1016/j.energy.2020.118059. ISSN 0360-5442.
- [26] W. Qiang, L. Yanzhong, W. Jiang, Analysis of power cycle based on cold energy of liquefied natural gas and low-grade heat source, Appl. Therm. Eng. 24 (4) (2004) 539–548, https://doi.org/10.1016/j.applthermaleng.2003.09.010.
- [27] T. He, Z.R. Chong, J. Zheng, Y. Ju, P. Linga, LNG cold energy utilization: prospects and challenges, Energy 170 (2019) 557–568, https://doi.org/10.1016/j. energy.2018.12.170.
- [28] K.A. Al-attab, Z.A. Zainal, Externally fired gas turbine technology: a review, Appl. Energy 138 (2015) 474–487, https://doi.org/10.1016/j.apenergy.2014.10.049.
- [29] M. Hou, Z. Wu, G. Yu, J. Hu, E. Luo, A thermoacoustic stirling electrical generator for cold exergy recovery of liquefied nature gas, Appl. Energy 226 (2018) 389–396, https://doi.org/10.1016/j.apenergy.2018.05.120.
- [30] X. Shi, D. Che, A combined power cycle utilizing low-temperature waste heat and LNG cold energy, Energy Convers. Manage. 50 (3) (2009) 567–575, https://doi. org/10.1016/j.enconman.2008.10.015.
- [31] M. Mehrpooya, B. Golestani, S.M. Ali Mousavian, Novel cryogenic argon recovery from the air separation unit integrated with LNG regasification and CO2 transcritical power cycle, Sustain. Energy Technol. Assess. 40 (2020) 100767, https://doi.org/10.1016/j.seta.2020.100767.
- [32] D.S. Ayou, V. Eveloy, Energy, exergy and exergoeconomic analysis of an ultra lowgrade heat-driven ammonia-water combined absorption power-cooling cycle for district space cooling, sub-zero refrigeration, power and LNG regasification, Energy Convers. Manage 213 (2020) 112790, https://doi.org/10.1016/j. enconman.2020.112790.
- [33] B. Ghorbani, A. Ebrahimi, M. Ziabasharhagh, Novel integrated CCHP system for generation of liquid methanol, power, cooling and liquid fuels using Kalina power cycle through liquefied natural gas regasification, Energy Convers. Manage 221 (2020) 113151, https://doi.org/10.1016/j.enconman.2020.113151.
- [34] H. Yu, T. Gundersen, E. Gençer, Optimal liquified natural gas (LNG) cold energy utilization in an Allam cycle power plant with carbon capture and storage, Energy Convers. Manage. 228 (2021) 113725, https://doi.org/10.1016/j. enconman.2020.113725.
- [35] J. Bao, T. Yuan, L. Zhang, N. Zhang, X. Zhang, G. He, Comparative study of liquefied natural gas (LNG) cold energy power generation systems in series and parallel, Energy Convers. Manage. 184 (2019) 107–126, https://doi.org/10.1016/ j.enconman.2019.01.040.
- [36] Z. Tian, Z. Qi, W. Gan, M. Tian, W. Gao, A novel negative carbon-emission, cooling, and power generation system based on combined LNG regasification and waste heat recovery: Energy, exergy, economic, environmental (4E) evaluations, Energy 257 (2022) 124528, https://doi.org/10.1016/j.energy.2022.124528.
- [37] A.H. Farajollahi, et al., Waste heat recovery of an UAV propulsion system based on PEM fuel cell by a novel transcritical CO 2 - LNG hybrid cycle; thermodynamic and multiple linear regression analyses, Energy Sources Part A Recov. Utiliz. Environ. Effects 44 (4) (2022) 8694–8717, https://doi.org/10.1080/ 15567036.2022.2123574.
- [38] Z. Tian, W. Zeng, B. Gu, Y. Zhang, X. Yuan, Energy, exergy, and economic (3E) analysis of an organic Rankine cycle using zeotropic mixtures based on marine engine waste heat and LNG cold energy, Energy Convers. Manage. 228 (2021) 113657, https://doi.org/10.1016/j.enconman.2020.113657.
- [39] Z. Yu, C. Feng, F. Bian, D. Wang, Investigation and optimization of a two-stage cascade ORC system for medium and low-grade waste heat recovery using liquefied natural gas cold energy, Int. J. Refriger. 135 (2022) 97–112, https://doi.org/ 10.1016/j.ijrefrig.2021.12.025.
- [40] J. Ma, X. Song, B. Zhang, N. Mao, T. He, Optimal design of dual-stage combined cycles to recover LNG cold energy and low-temperature waste thermal energy for sustainable power generation, Energy Convers. Manage. 269 (2022) 116141, https://doi.org/10.1016/j.enconman.2022.116141.
- [41] L.-H. Zhi, P. Hu, L.-X. Chen, G. Zhao, Thermodynamic analysis of an innovative transcritical CO2 parallel Rankine cycle driven by engine waste heat and liquefied natural gas cold, Energy Convers. Manage. 209 (2020) 112583, https://doi.org/ 10.1016/j.enconman.2020.112583.
- [42] S. Yao, C. Li, Y. Wei, Design and optimization of a zero carbon emission system integrated with the utilization of marine engine waste heat and LNG cold energy for LNG-powered ships, Appl. Therm. Eng. 231 (2023) 120976, https://doi.org/ 10.1016/j.applthermaleng.2023.120976.
- [43] T. Hai, et al., Optimization next to environmental analysis of harvesting waste heat from a biomass-driven externally-fired gas turbine cycle for sub-zero cooling and production of hydrogen, freshwater, and hot water, Appl. Therm. Eng. 223 (2023) 119884, https://doi.org/10.1016/j.applthermaleng.2022.119884.
- [44] O. Konur, C.O. Colpan, O.Y. Saatcioglu, A comprehensive review on organic Rankine cycle systems used as waste heat recovery technologies for marine applications, Energy Sources Part A Recov. Utiliz. Environ. Effects 44 (2) (2022) 4083–4122, https://doi.org/10.1080/15567036.2022.2072981.
- [45] C. Tian, C. Su, C. Yang, X. Wei, P. Pang, J. Xu, Exergetic and economic evaluation of a novel integrated system for cogeneration of power and freshwater using waste heat recovery of natural gas combined cycle, Energy 264 (2023) 126227, https:// doi.org/10.1016/j.energy.2022.126227.
- [46] S.-H. Cha, S.-I. Na, Y.H. Lee, M.S. Kim, Thermodynamic analysis of a gas turbine inlet air cooling and recovering system in gas turbine and CO2 combined cycle using cold energy from LNG terminal, Energy Convers. Manage 230 (2021) 113802, https://doi.org/10.1016/j.enconman.2020.113802.
- [47] B. Peris, J. Navarro-Esbrí, F. Mol´es, A. Mota-Babiloni, Experimental study of an ORC (organic Rankine cycle) for low grade waste heat recovery in a ceramic industry, Energy 85 (2015) 534–542. ISSN 0360-5442.
- [48] H. Jouhara, D. Bertrand, B. Axcell, L. Montorsi, M. Venturelli, S. Almahmoud, M. Milani, L. Ahmad, A. Chauhan, Investigation on a full-scale heat pipe heat exchanger in the ceramics industry for waste heat recovery, Energy 223 (2021) 120037. ISSN 0360-5442.
- [49] T.G.C. Veloso, C.A.R. Sotomonte, CJ.R. Coronado, MA.R. Nascimento, Multiobjective optimization and exergetic analysis of a low-grade waste heat recovery ORC application on a Brazilian FPSO, Energy Convers. Manage. 174 (2018) 537–551. ISSN 0196-8904.
- [50] Dwivedi, A. and Mishra, R.S., Analysis of organic Rankine cycle with waste heat recovery from gas power plant using loop heat pipes. J. Mech. Eng. Res. Dev., ISSN, pp.1024-1752.
- [51] Z. Tian, Y. Yue, B. Gu, W. Gao, Y. Zhang, Thermo-economic analysis and optimization of a combined Organic Rankine Cycle (ORC) system with LNG cold
- energy and waste heat recovery of dual-fuel marine engine, Int. J. Energy Res. 44 (2020) 9974–9994.
- [52] B. Jiang, D. Xia, H. Zhang, H. Pei, X. Liu, Effective waste heat recovery from industrial high-temperature granules: a moving bed indirect heat exchanger with embedded agitation, Energy 208 (2020) 118346. ISSN 0360-5442.
- [53] A.M. Alsayah, JJ. Faraj, AA. Eidan, The augmentation of the heat recovery by using evaporative cooling in HVAC applications: experimental study, Int. J. Thermofluids 22 (2024) 100671. ISSN 2666-2027.
- [54] D. Brough, J. Ramos, B. Delpech, H. Jouhara, Development and validation of a TRNSYS type to simulate heat pipe heat exchangers in transient applications of waste heat recovery, Int. J. Thermofluids 9 (2021) 100056. ISSN 2666-2027.
- [55] A.I. Turja, K.N. Sadat, Y. Khan, M. Monjurul Ehsan, Cascading the transcritical CO2 and organic Rankine cycles with supercritical CO2 cycles for waste heat recovery, Int. J. Thermofluids 20 (2023) 100508. ISSN 2666-2027.
- [56] A.S. Chowdhury, M.M. Ehsan, A critical overview of working fluids in organic Rankine, supercritical rankine, and supercritical brayton cycles under various heat grade sources, Int. J. Thermofluids 20 (2023) 100426. ISSN 2666-2027.
- [57] A.E. Elahi, T. Mahmud, M. Alam, J. Hossain, B.N. Biswas, Exergy analysis of organic Rankine cycle for waste heat recovery using low GWP refrigerants, Int. J. Thermofluids 16 (2022) 100243. ISSN 2666-2027.
- [58] M. Mahmoud, S. Naher, M. Ramadan, M.A. Abdelkareem, H. Jaber, A.-G. Olabi, Investigation of a ground-cooled organic Rankine cycle for waste heat recovery, Int. J. Thermofluids 18 (2023) 100348. ISSN 2666-2027.
- [59] AR. Imre, AM. Ahmed, Effect of the working fluids critical temperature on thermal performance for trilateral flash cycle and organic Rankine cycle, Int. J. Thermofluids 20 (2023) 100417. ISSN 2666-2027.
- [60] Xu Zheng, Y. Li, Ji Zhang, Z. Zhang, C. Guo, N. Mei, Design and multi-objective optimization of combined air separation and ORC system for harnessing LNG cold energy considering variable regasification rates, Int. J. Hydrogen. Energy 57 (2024) 210–223. ISSN 0360-3199.
- [61] Y.A.A. Laouid, C. Kezrane, Y. Lasbet, Apostolos Pesyridis, Towards improvement of waste heat recovery systems: A multi-objective optimization of different organic Rankine cycle configurations, Int. J. Thermofluids 11 (2021) 100100. ISSN 26662027.
- [62] M. Taal, I. Bulatov, J. Klemes, P. Stehlik, Cost estimation and energy price forecasts for economic evaluation of retrofit projects, Appl. Therm. Eng. 23 (2003) 1819–1835.
- [63] A.C. Caputo, P.M. Pelagagge, P. Salini, Heat exchanger design based on economic optimization, Appl. Therm. Eng. 28 (2008) 1151–1159.
- [64] M.A.A Sadreddini, M. Fani, A. Mohammadi, Thermodynamic analysis of a new cascade ORC and transcritical CO2 cycle to recover energy from medium temperature heat source and liquefied natural gas, Energy Convers. Manage 167 (2018) 9–20, https://doi.org/10.1016/j.enconman.2018.04.093.
저작권 및 참고 자료
본 자료는 Rizvi Arefin Rinik 외 저자의 논문 "Design of gravity assisted heat exchanger and its application on enhanced waste heat recuperation utilizing organic Rankine and LNG system"을 기반으로 작성되었습니다.
논문 출처: https://doi.org/10.1016/j.ijft.2024.100822
본 자료는 위 논문을 바탕으로 요약 작성되었으며, 상업적 목적으로 무단 사용이 금지됩니다.
Copyright © 2025 CASTMAN. All rights reserved.