本紹介資料は、「Metals (MDPI)」によって発行された論文「Experimental and Numerical Study of an Automotive Component Produced with Innovative Ceramic Core in High Pressure Die Casting (HPDC)」に基づいています。
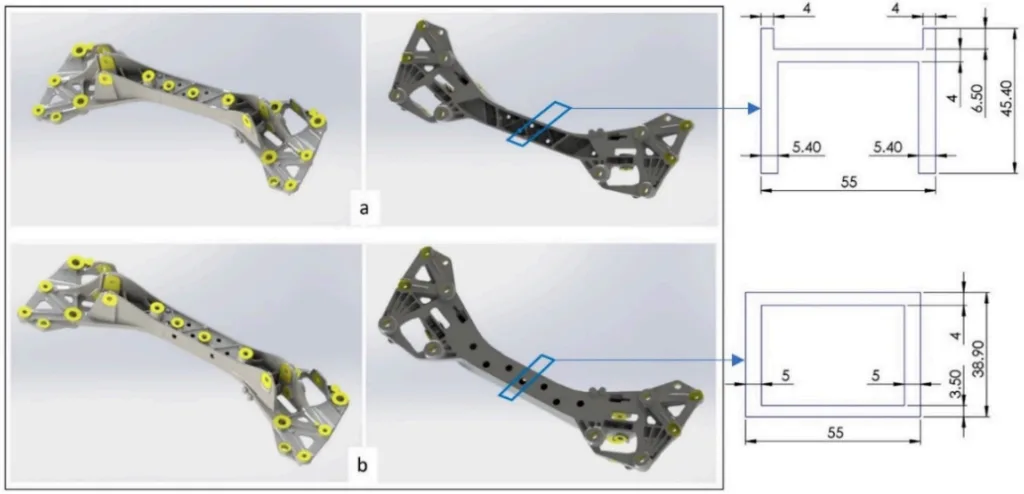
1. 概要:
- 論文タイトル: Experimental and Numerical Study of an Automotive Component Produced with Innovative Ceramic Core in High Pressure Die Casting (HPDC)
- 著者: Giovanna Cornacchia, Daniele Dioni, Michela Faccoli, Claudio Gislon, Luigi Solazzi, Andrea Panvini and Silvia Cecchel
- 発行年: 2019
- 発行学術誌/学会: Metals (MDPI)
- キーワード: non-ferrous alloys; ceramic core; FEA; HPDC; de-coring; material characterization (非鉄合金; セラミックコア; 有限要素解析; 高圧ダイカスト; コア除去; 材料特性評価)
2. 抄録:
軽量化と材料置換は、自動車産業におけるトレンドとなっています。高圧ダイカスト(HPDC)は、軽合金の大量生産のための従来の鋳造技術であり、最近では複雑で薄肉形状の自動車部品など、重要部品の製造に広く応用されています。しかし、この手頃な技術の主な制約は、中空断面やアンダーカットを持つ部品の設計と実現が困難であることです。HPDCの競争力をさらに高める革新的な方法は、HPDCで使用される高圧に耐えることができる新しい消失コア(lost core)を使用して、複雑なアンダーカット形状の部品を成形することです。本論文では、HPDCによる乗用車用アルミニウムクロスメンバーの製造における革新的なセラミック消失コアの使用を調査します。まず、クロスメンバーの設計を改善し、技術的特徴を確認するために、プロセスおよび構造シミュレーションを実施しました。その結果に基づき、プロセスパラメータを選択し、最終的に特性評価を行うプロトタイプをいくつか製造しました。これらの分析は、セラミックコアを用いたHPDCによる中空部品の製造の実現可能性を示しています。
3. 緒言:
自動車産業では、車両性能の向上、燃費の削減、排出ガスの低減のために、軽量部品の需要が高まっています。高圧ダイカスト(HPDC)は、大量生産、低コスト、ニアネットシェイプのアルミニウム部品に適した競争力のある技術です。しかし、HPDCは従来、複雑な中空断面やアンダーカットを持つ部品の製造には課題がありました。これらはしばしば金属製の可動コアを必要とし、重力鋳造で使用される消失コアと比較して設計の自由度が制限されます。この制限を克服するためには、HPDCの高圧および高速流動に対応できる消失コアが必要です。HPDC用のソルトコアに関する研究は存在しますが、課題は残っています。本論文では、複雑な形状と良好な公差のために射出成形によって製造され、高いHPDC圧力(1000 bar以上)に耐え、ガスを放出しない革新的なセラミック消失コアの使用を探求します。この研究は、これらのセラミックコアを使用してHPDCで乗用車用アルミニウムクロスメンバーを再設計、製造し、その実現可能性を実証することに焦点を当てています。目標は、従来の方法と比較して、軽量化、ねじり剛性の向上、製造時間の短縮といった利点を達成することです。
4. 研究概要:
研究テーマの背景:
自動車分野における軽量化の推進は、アルミニウムのような軽合金を有利にしています。HPDCは、その高い生産性と費用対効果から、これらの合金の主要な製造方法です。しかし、重量比剛性に優れた複雑な中空部品は、従来のHPDC法(金属コア使用)ではアンダーカットや内部キャビティの組み込みが困難であるか、従来の消失コア(例:砂、塩)が高い射出圧力と互換性がないため、製造が困難です。
先行研究の状況:
先行研究では、鋳造プロセス用に様々な消耗性コア(砂、塩、金属、有機材料)が検討されてきました。特にHPDC用途ではソルトコアが注目され、その強度やプロセスパラメータに関する研究が行われています。シリンダーブロックなどの部品にHPDCで可溶性コアを使用する特許も存在します。しかし、要求される強度とプロセスの信頼性を達成することは依然として課題です。射出成形によって製造されるセラミックコアは、良好な寸法公差、低い表面粗さ、鋳造中のガス放出がないといった潜在的な利点を提供し、より高い機械的特性をもたらす可能性があります。リーチングや高圧ウォータージェットのようなコア除去方法が存在しますが、複雑な内部キャビティから抵抗性のあるコアを除去することは考慮が必要です。
研究目的:
主な目的は、革新的なセラミック消失コアを使用してHPDC法で複雑な中空自動車部品(アルミニウム製乗用車クロスメンバー)を製造することの実現可能性を調査し、実証することでした。これには、部品の再設計、プロセスと構造性能のシミュレーション、プロトタイプの製造、コアと最終鋳造部品の両方の特性評価が含まれます。
コア研究内容:
本研究では、既存の開断面アルミニウムクロスメンバー(EN AC-43500合金)を、セラミックコア(Al2O3 + SiO2 + K2Oベース)を使用して閉断面ボックス形状に再設計しました。有限要素解析(FEA)を用いて、元の設計と修正された設計の構造性能(モード解析、座屈)を比較しました。計算流体力学(CFD)シミュレーションを実施し、セラミックコアの熱特性を考慮して、両方の設計についてHPDCプロセス(充填、凝固、空気巻き込み)を分析しました。実験作業には、異なる焼結温度で製造されたセラミックコアの特性評価(密度、コア除去方法、3点曲げ試験による機械的特性)が含まれました。選択されたコアタイプを使用してHPDCプロトタイプを製造しました。最後に、鋳造されたプロトタイプは、微細構造解析(OM、SEM/EDS)およびビッカース微小硬さ試験によって特性評価されました。
5. 研究方法論
研究設計:
本研究は、数値シミュレーションと実験的検証を組み合わせたアプローチを採用しました。既存の自動車クロスメンバー設計を、セラミックコアによって可能になる中空断面を組み込むように修正しました。元の開断面設計と新しい閉断面(ボックス)設計の間で比較分析(数値的および暗黙的な実験的)を行いました。研究は、部品再設計 -> 数値シミュレーション(構造FEAおよびプロセスCFD) -> セラミックコア材料の選択と特性評価 -> HPDCによるプロトタイプ製造 -> 最終部品特性評価の順序で進められました。
データ収集および分析方法:
- 数値シミュレーション:
- 構造解析 (FEA): Autodesk Simulation® ソフトウェアを使用し、放物線ブリック要素で実施。自由-自由および固定境界条件下でのモード解析(固有振動数)、および印加された力およびモーメント荷重下での座屈解析を実施。
- プロセスシミュレーション (CFD): PiQ2のCastleBodyソフトウェアを使用し、圧縮性-非圧縮性二相流体体積(VOF)定式化を採用して実施。シミュレーションでは、アルミニウム合金(EN AC-43500)とセラミックコアインサートの両方の熱特性を考慮し、金型充填、凝固、空気巻き込み、および潜在的な欠陥を分析。局所的な詳細化を伴う六面体メッシュ(約1,000,000セル)を使用。
- 実験的特性評価:
- セラミックコア: エタノールを用いたピクノメーターで密度を測定。化学的リーチング(各種酸)および高圧ウォータージェットを用いてコア除去効果をテスト。UNI EN 843-1およびUNI EN 843-3に従って、3点曲げ試験(Instron 3369)により機械的特性(曲げ強度σf、ヤング率E)を決定。
- HPDC部品: IDRA OLS2000 HPDCマシンでプロトタイプを製造。光学顕微鏡(OM - Leica DMI 5000M)および走査型電子顕微鏡(SEM - LEO EVO 40)とエネルギー分散型分光法(EDS - Link Analytical eXL)を用いて横断面の微細構造を分析。ASTM E92-16およびASTM E140-02に従って、Micro Duromat 4000 Reichert Jung装置を用いて断面全体のビッカース微小硬さ(HV0.3)を測定。
研究テーマと範囲:
本研究は、自動車構造部品(クロスメンバー)のためのHPDCプロセスにおける革新的なセラミック消失コアの適用に焦点を当てました。研究範囲は以下の通りです:
- クロスメンバーの幾何学的再設計(開断面から閉断面へ)。
- 比較構造性能分析(剛性、座屈抵抗)。
- 流体流動、熱伝達、凝固、および欠陥予測(空気巻き込み、コールドジョイント、収縮)を含むHPDCプロセス実現可能性シミュレーション。
- Al2O3 + SiO2 + K2Oベースのセラミックコアの特性評価(物理的、コア除去のための化学的耐性、機械的特性)。
- EN AC-43500アルミニウム合金を用いたプロトタイプの実験的製造。
- アルミニウム合金と残留セラミック/サーメット材料間の界面を含む、最終鋳造部品の冶金学的特性評価。
6. 主要な結果:
主要な結果:
- 閉断面ボックス形状に再設計されたクロスメンバーは、元の開断面プロファイルと比較してわずかな重量削減を達成しました(3.93 kg 対 3.95 kg)。
- FEAシミュレーションにより、新しい設計は元の設計と比較して優れた構造性能を示すことが実証されました:より高い固有振動数(剛性の向上を示す、例:自由-自由条件下での最初のねじり振動数が約54.3%増加)、および力とモーメントの両方の荷重条件下で著しく高い座屈荷重係数。
- CFDプロセスシミュレーションは、セラミックコアを用いたHPDCプロセスの実現可能性を確認しました。予測された鋳造品質(空気巻き込み、凝固パターン、潜在的欠陥)は、コアのない従来のHPDCプロセスと非常に類似していました。シミュレーションパラメータ(例:充填時間約0.2秒、凝固時間約8.4秒)は、標準的なHPDCの実践と一致していました。
- セラミックコアの特性評価では、密度が約1.92-1.98 g/cm³であることが示されました。化学的リーチング試験では、HF溶液のみがセラミックをエッチングしましたが、これらはアルミニウムに深刻な損傷を与えました。高圧ウォータージェットが効果的で損傷を与えないコア除去方法として特定されました。機械的試験(3点曲げ)では、曲げ強度とヤング率が焼結温度とともに増加することが示され、プロトタイプには「c-1110 °C」バリアントが選択されました。
- 選択されたセラミックコアを使用して、約100個の中空クロスメンバープロトタイプがHPDCで成功裏に製造され、その後ウォータージェットでコアが除去されました。製造サイクルタイムは約70秒でした。
- プロトタイプの微細構造分析により、EN AC-43500合金の予想される構造(α-Alマトリックス、Al-Si共晶、α-Al15(Mn,Fe)3Si2のような金属間化合物)が明らかになりました。一部の領域でアルミニウムとセラミックコアの界面に薄い(約100 µm)サーメット層(セラミック-金属混合物)が観察されましたが、アルミニウムとの良好な密着性を示し、用途に有害とは見なされませんでした。
- 部品断面全体のビッカース微小硬さ測定では、75-90 HV0.3の範囲の値が得られ、これはこの合金とプロセスにとって典型的であり、コアと薄いサーメット層の存在がバルク材料全体の機械的特性に悪影響を与えないことを示しています。
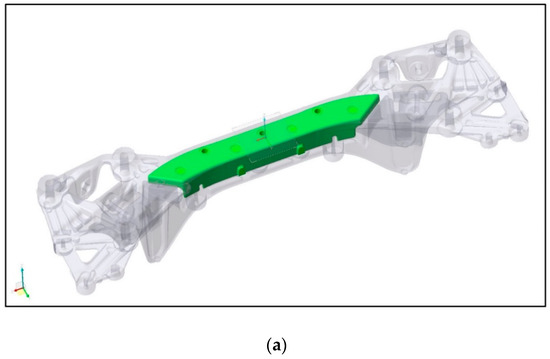
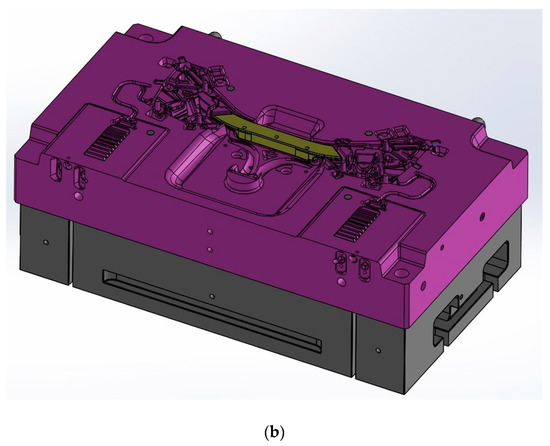
Figure 2. (a) 3D model of the closed profile crossbeam with ceramic core. (b) The geometry and the positioning solutions in the mold cavity adopted for the ceramic core.
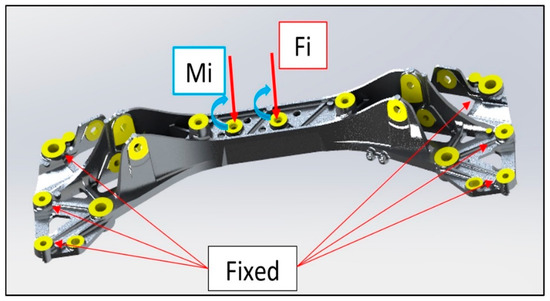
Figure 3. Buckling analysis model: boundary condition and load cases.Table 2. FEM simulation parameters.
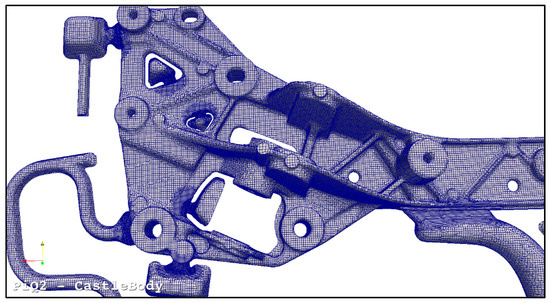
Figure 4. Local mesh refinement.
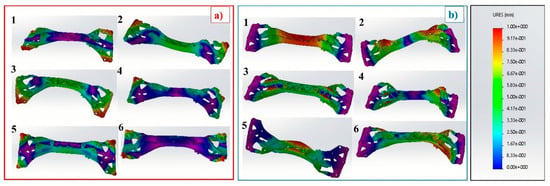
Figure 5. Displacement trend for the first six vibration modes for original geometry: (a) free-free boundary conditions and (b) fixed conditions.
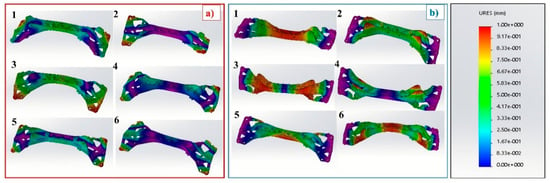
Figure 6. Displacement trend for the first six vibration modes for new geometry: (a) free-free boundary conditions and (b) fixed conditions.
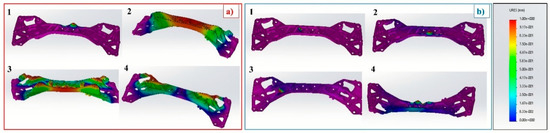
Figure 7. Displacement trend for the first four deformations for original geometry: (a) load case 1 (F); (b) load case 2 (M).
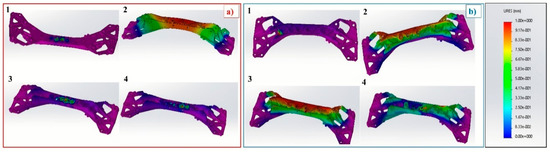
Figure 8. Displacement trend for the first four deformations for new geometry: (a) load case 1 (F); (b) load case 2 (M).
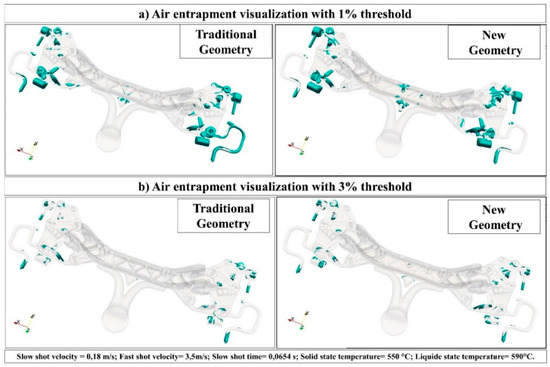
Figure 9. Air entrapment visualization (a) with 1% threshold and (b) with 3% threshold for traditional and new geometry.
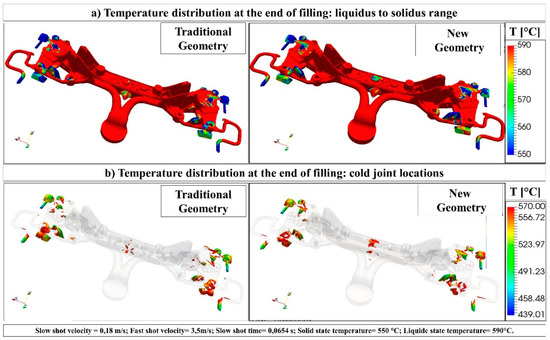
Figure 10. (a) Temperature distribution at the end of filling: liquidus to solidus range; (b) Temperature distribution at the end of filling: cold joint locations.
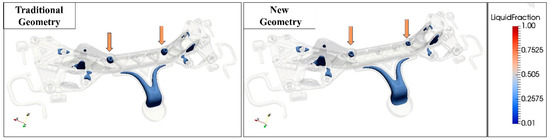
Figure 11. Results of solidification analysis.Figure 10a,b shows the temperature distribution of the alloy at the end of filling in the range between liquidus and solidus temperatures.
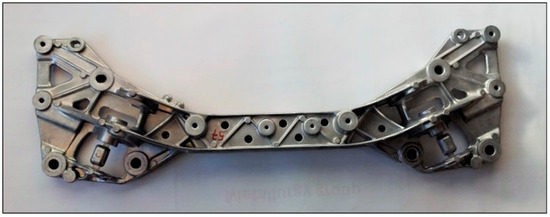
Figure 12. Hollowed aluminum high pressure die casting component.
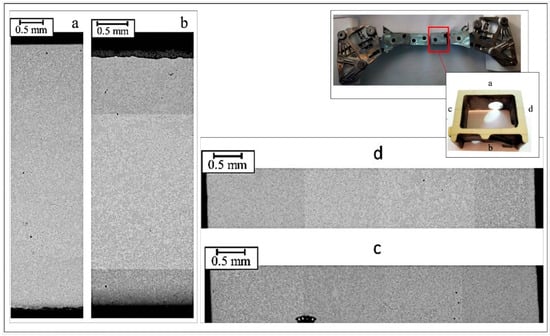
Figure 13. Collage of various micrographs of the transverse sections of the hollowed crossbeam.
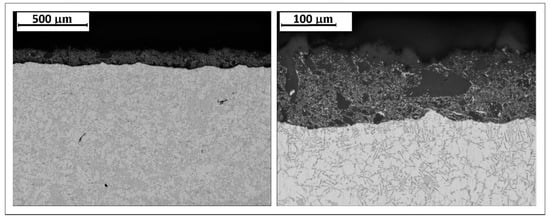
Figure 14. Optical microscope details of the transverse sections of the hollowed crossbeam.
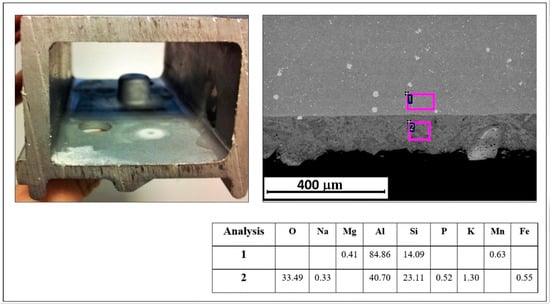
Figure 15. Visual inspection on cermet and SEM image with results of EDS analysis (wt%).
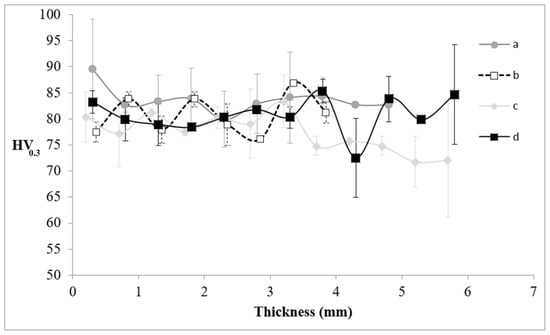
Figure 16. Vickers microhardness HV0.3 variation with respect to the thickness along the transverse section of .
図リスト:
- Figure 1. 3D design and schematic sketch of the section profile of (a) original crossbeam (b) modified crossbeam. The dimensions are expressed in (mm).
- Figure 2. (a) 3D model of the closed profile crossbeam with ceramic core. (b) The geometry and the positioning solutions in the mold cavity adopted for the ceramic core.
- Figure 3. Buckling analysis model: boundary condition and load cases.
- Figure 4. Local mesh refinement.
- Figure 5. Displacement trend for the first six vibration modes for original geometry: (a) free-free boundary conditions and (b) fixed conditions.
- Figure 6. Displacement trend for the first six vibration modes for new geometry: (a) free-free boundary conditions and (b) fixed conditions.
- Figure 7. Displacement trend for the first four deformations for original geometry: (a) load case 1 (F); (b) load case 2 (M).
- Figure 8. Displacement trend for the first four deformations for new geometry: (a) load case 1 (F); (b) load case 2 (M).
- Figure 9. Air entrapment visualization (a) with 1% threshold and (b) with 3% threshold for traditional and new geometry.
- Figure 10. (a) Temperature distribution at the end of filling: liquidus to solidus range; (b) Temperature distribution at the end of filling: cold joint locations.
- Figure 11. Results of solidification analysis.
- Figure 12. Hollowed aluminum high pressure die casting component.
- Figure 13. Collage of various micrographs of the transverse sections of the hollowed crossbeam.
- Figure 14. Optical microscope details of the transverse sections of the hollowed crossbeam.
- Figure 15. Visual inspection on cermet and SEM image with results of EDS analysis (wt%).
- Figure 16. Vickers microhardness HV0.3 variation with respect to the thickness along the transverse section of Figure 13.
7. 結論:
本研究は、革新的なセラミック消失コアを使用してHPDC法により、より軽量で構造的に改善された安全関連自動車部品(アルミニウムクロスメンバー)を製造することの実現可能性を成功裏に実証しました。再設計されたボックス形状は、元の開断面設計と比較して、動的挙動(より高い固有振動数)と座屈に対する抵抗性が大幅に向上していることを示しました。プロセスシミュレーションは、セラミックコアを用いたHPDCプロセスが従来のプロセスと同等の鋳造品質を達成できることを確認しました。実験的な特性評価により、適切なセラミックコア特性と効果的なコア除去方法(高圧ウォータージェット)が特定されました。製造されたプロトタイプの分析により、コアと金属の界面に薄く、有害ではないサーメット層が存在するにもかかわらず、微細構造と硬度が標準的なHPDC部品と一致していることが確認されました。この結果は、セラミック消失コアを用いたHPDCが、複雑で中空、ニアネットシェイプの部品を大量に製造するための優れた可能性を秘めていることを示しており、先進的な自動車用途におけるHPDCの競争力を高め、中空構造のさらなる軽量化と設計最適化の可能性を開くものです。
8. 参考文献:
- [1] Chindamo, D.; Gadola, M. Reproduction of real-world road profiles on a four-poster rig for indoor vehicle chassis and suspension durability testing. Adv. Mech. Eng. 2017, 9, 1–10. [CrossRef]
- [2] Chiaberge, M. New Trends and Developments in Automotive Industry; InTech: London, UK, 2011; ISBN 978-953-307-999-8. [CrossRef]
- [3] Tempelman, E. Multi-Parametric study of the effect of materials substitution on life cycle energy use and waste generation of passenger car structures. Transp. Res. Part D Transp. Environ. 2011, 16, 479–485. [CrossRef]
- [4] Cecchel, S.; Chindamo, D.; Turrini, E.; Carnevale, C.; Cornacchia, G.; Gadola, M.; Panvini, A.; Volta, M.; Ferrario, D.; Golimbioschi, R. Impact of reduced mass of light commercial vehicles on fuel consumption, CO2 emissions, air quality, and socio-economic costs. Sci. Total Environ. 2018, 613–614, 409–417. [CrossRef] [PubMed]
- [5] Cecchel, S.; Ferrario, D.; Panvini, A.; Cornacchia, G. Lightweight of a cross beam for commercial vehicles: Development, testing and validation. Mater. Des. 2018, 149, 122–134. [CrossRef]
- [6] Cecchel, S.; Ferrario, D. Numerical and experimental analysis of a high pressure die casting Aluminum suspension cross beam for light commercial vehicles. La Metallurgia Italiana 2016, 6, 41–44.
- [7] Morello, L.; Rosti Rossini, L.; Pia, G.; Tonoli, A. The Automotive Body: Volume I: Components Design; Springer: New York, NY, USA, 2001; ISBN 978-94-007-0513-5.
- [8] Cecchel, S.; Cornacchia, G.; Panvini, A. Cradle-to-Gate Impact Assessment of a High-Pressure Die-Casting Safety-Relevant Automotive Component. JOM 2016, 8, 2443–2448. [CrossRef]
- [9] Cecchel, S.; Chindamo, D.; Collotta, M.; Cornacchia, G.; Panvini, A.; Tomasoni, G.; Gadola, M. Lightweighting in light commercial vehicles: Cradle-to-grave life cycle assessment of a safety relevant component. Int. J. Life Cycle Assess. 2018, 23, 1–12. [CrossRef]
- [10] Cecchel, S.; Collotta, M.; Cornacchia, G.; Panvini, A.; Tomasoni, G. A comparative cradle-to gate impact assessment: Primary and secondary aluminum automotive components case. La Metallurgia Italiana 2018, 2, 46–55.
- [11] Cecchel, S.; Cornacchia, G.; Gelfi, M. Corrosion behavior of primary and secondary AlSi High Pressure Die Casting alloys. Mater. Corros. 2017, 68, 961–969. [CrossRef]
- [12] Solazzi, L. Applied research for Weight Reduction of an industrial Trailer. FME Trans. 2012, 40, 57–62.
- [13] Solazzi, L. Wheel rims for industrial vehicles: Comparative and experimental analyses. Int. J. Heavy Veh. Syst. 2011, 18, 214–225. [CrossRef]
- [14] Hirsch, J. Aluminium in Innovative Light-Weight Car Design. Mater. Trans. 2011, 52, 818–824. [CrossRef]
- [15] Chindamo, D.; Lenzo, B.; Gadola, M. On the vehicle sideslip angle estimation: A literature review of methods, models and innovations. Appl. Sci. 2018, 8, 355. [CrossRef]
- [16] Henriksson, F.; Johansen, K. On Material Substitution in Automotive BIWs – From Steel to Aluminum Body Sides. Procedia CIRP 2016, 50, 683–688. [CrossRef]
- [17] Kelkar, A.; Roth, R.; Clark, J. Automobile Bodies: Can Aluminum Be an Economical Alternative To Steel? JOM 2001, 53, 28–32.
- [18] Zhoua, J.; Wana, X.; Lia, Y. Advanced aluminium products and manufacturing technologies applied on vehicles at the EuroCarBody conference. Mater. Proc. 2015, 2, 5015–5022. [CrossRef]
- [19] Fridlyander, I.N.; Sister, V.G.; Grushko, O.E.; Berstenev, V.V.; Sheveleva, L.M.; Ivanova, L.A. Aluminum alloys: Promising materials in the automotive industry. Met. Sci. Heat Treat. 2002, 44, 365–370. [CrossRef]
- [20] Dioni, D.; Cecchel, S.; Cornacchia, G.; Faccoli, M.; Panvini, A. Effects of artificial aging conditions on mechanical properties of gravity cast B356 aluminum alloy. Trans. Nonferrous Met. Soc. China 2015, 25, 1035–1042. [CrossRef]
- [21] Faccoli, M.; Dioni, D.; Cecchel, S.; Cornacchia, G.; Panvini, A. Optimization of heat treatment of gravity cast Sr-modified B356 aluminum alloy. Trans. Nonferrous Met. Soc. China 2017, 27, 1698–1706. [CrossRef]
- [22] Haracopos, B.; Fisher, T.P. The Technology of Gravity Die Casting; Hart Pub. Co.: Oxford, UK, 1968.
- [23] ASM. Metals Handbook, 10th ed.; ASM-Metals Park: Geauga, OH, USA, 1990.
- [24] Campbell, J.; Harding, R.A. Casting Technology; TALAT 2.0 [CD-ROM]; EAA: Bruxelles, Belgium, 2000.
- [25] Schleg, S.; Kamicki, D.P. Guide to casting and moulding processes. Engineered casting solutions. Technical Articles, 2000.
- [26] Brown, J.R. Foseco Non-Ferrous Foundryman’s Handbook; Elsevier: Oxford, UK, 1999; ISBN 9780080531878.
- [27] Street, A.C. The Diecasting Book, 2nd ed.; Portcullis Press: London, UK, 1990.
- [28] Nagendra Parashar, S.; Mittal, R.K. Elements of Manufacturing Processes; PHI learning Pvt. Ltd.: New Delhi, India, 2006.
- [29] Vinarcik, E.J. High Integrity Die Casting Processes; John Wiley & Sons: Hoboken, NJ, USA, 2002; ISBN 978-0-471-20131-1.
- [30] Andresen, W. Die Cast Engineering: A Hydraulic, Thermal, and Mechanical Process; CRC Press: Boca Raton, FL, USA, 2004.
- [31] Jelínek, P.; Adámková, E.; Mikšovský, F.; Beňo, J. Advances in technology of soluble cores for die castings. Arch. Foundry Eng. 2015, 15, 29–34. [CrossRef]
- [32] Pierri, D. Lost Core: New Perspectives in Die Casting. Available online: https://www.buhlergroup.com/northamerica/en/industry-solutions/die-casting/latest-news/details-7797.htm?title= (accessed on 9 February 2019).
- [33] Czerwinski, F.; Birsan, G.; Benkel, F.; Kasprzak, W.; Walker, M.J.; Smith, J.; Trinowski, D.; Musalem, I. Developing strong core technology for high pressure die casting. Automot. Mater. 2017, 8, 1–11.
- [34] Rupp, S.; Heppes, F. La rivoluzione nella pressofusione. Tecnico-Industria Fusoria 2017, 4, 72–76.
- [35] Donahue, R.J.; Degler, M.T. Congruent Melting Salt Alloys for Use as Salt Cores in High Pressure Die Casting. U.S Patent US9527131B1, 25 August 2014.
- [36] Radadiya, V.A.; Dave, K.G.; Patel, K.R. Design and analysis of salt core for a casting of alluminium alloys. Int. J. Adv. Eng. Res. Dev. 2015, 2, 344–348.
- [37] Jelinek, P.; Miksovsky, F.; Beoo, J.; Adamkova, E. Development of foundry cores based on inorganic salts. MTAEC9 2013, 47, 689–693.
- [38] Jelínek, P.; Adámková, E. Lost cores for high-pressure die casting. Arch. Foundry Eng. 2014, 14, 101–104. [CrossRef]
- [39] Yaokawa, J.; Miura, D.; Anzai, K.; Yamada, Y.; Yoshii, H. Strength of salt core composed of alkali carbonate and alkali chloride mixtures made by casting technique. Mater. Trans. 2007, 8, 1034–1041. [CrossRef]
- [40] Yaokawa, J.; Koichi, A.; Yamada, Y.; Yoshii, H. Strength of salt core for die casting. In Proceedings of the International Conference CastExpo ’05 NADCA, St. Louis, MO, USA, 16–19 April 2005; NADCA: St. Louis, MO, USA, 2005.
- [41] Fuchs, B.; Eibisch, H.; Körner, C. Core viability simulation for salt core technology in high-pressure die casting. Int. J. Metalcast. 2013, 7, 39–45. [CrossRef]
- [42] Fuchs, B.; Körner, C. Mesh resolution consideration for the viability prediction of lost salt cores in the high pressure die casting process. Prog. Comput. Fluid Dyn. 2014, 14, 24–30. [CrossRef]
- [43] Mizukusa, Y. Casting Apparatus and Casting Method for Producing Cylinder Block. U.S. Patent 5,690,159, 27 August 1996.
- [44] Ackerman, A.D.; Aula, H.A. Method of Making a Cast Aluminum Based Engine Block. U.S. Patent 4,446,906, 8 May 1984.
- [45] Brown, W.N.; Robinson, P.M. Soluble Metal Casting Cores Comprising a Water Soluble Salt and a Synthetic Resin. U.S. Patent 364,549, 22 July 1969.
- [46] Gibbons, W.A. Core or Filler of Fusible Material for Hollow Vulcanizable Articles. U.S. Patent 1,523,519, 20 January 1925.
- [47] Foreman, R.W. Mixture and Method for Preparing Casting Cores and Cores Prepared Thereby. U.S. Patent 4,840,219, 20 June 1989.
- [48] Sakoda, T. Water Soluble Core for Pressure Die Casting and Process for Making the Same. U.S. Patent 3,963,818, 15 June 1976.
- [49] Molina, R.; Moschini, R. Production of hollow components in high pressure die casting through the use of ceramic lost cores. In Proceedings of the International Conference High Tech Die Casting 2012, Vicenza, Italy, 9–10 April 2012.
- [50] Moschini, R.; Calzolaro, A.L. Method for Manufacturing Monolithic Hollow Bodies by Means of a Casting or Injection Moulding Process. Patent International Publication No WO 2011/061593 A1, 26 May 2011.
- [51] Solazzi, L. Innovative Bolted junction with high ductility for circular tubular element. J. Constr. Steel Res. 2015, 112, 175–182. [CrossRef]
- [52] Mats, G.; Larson, F.B. The Finite Element Method: Theory, Implementation and Applications; Springer: Berlin, Germany, 2010; ISBN 978-3-642-33286-9.
- [53] Dimitrios, G. Pavlou Essentials of Finite Element Method for Mechanical and Industrial Engineers; Elsevier: Amsterdam, The Netherlands, 2015; ISBN 978-0-12-802386-0.
- [54] Panvini, A.; Molin, D.; Gislon, C. Dual phase simulation for high pressure die casting: Overview and validation of its capabilities. In Proceedings of the HTDC Conference 2016, AIM, Venice, Italy, 22–23 June 2016.
- [55] Panvini, A.; Gislon, C. Air entrapment prediction in diecasting through dual phase simulation. In Proceedings of the Die Casting Congress Tabletop 2013, Louisville, KY, USA, 16–18 September 2013.
- [56] Miller, A. PQ2 and Gating Manual; NADCA: Arlington Heights, IL, USA, 2016.
- [57] UNI EN 843-1:2007. Advanced Technical Ceramics—Mechanical Properties of Monolithic Ceramics at Room Temperature—Part 1: Determination of Flexural Strength; NSAI: Dublin, Ireland, 2007.
- [58] UNI EN 843-2:2007. Advanced Technical Ceramics—Mechanical Properties of Monolithic Ceramics at Room Temperature—Part 2: Determination of Young’s Modulus, Shear Modulus and Poisson’s Ratio; NSAI: Dublin, Ireland, 2007.
- [59] Smith, J.H. An Introduction to Modern Vehicle Design; Elsevier: Oxford, UK, 2002; ISBN 07506 5044 3.
- [60] Pacejka, H.B. Tyre and Vehicle Dynamics, 2nd ed.; Butterworth-Heinemann: Oxford, UK, 2006; ISBN 0-7506-6918-7.
- [61] Franke, R.; Dragulin, D.; Zovi, A.; Casarotto, F. Progress in ductile aluminium high pressure die casting alloys for the automotive industry. La Metallurgia Italiana 2007, 5, 21–26.
- [62] Tinklepaugh, J.R.; James, R. Cermets; Reinhold Publishing Corporation: New York, NY, USA, 1960; ASIN B0007E6FO4.
- [63] Hanaor, D.A.H.; Hu, L.; Kan, W.H.; Proust, G.; Foley, M.; Karaman, I.; Radovic, M. Compressive performance and crack propagation in Al alloy/Ti2AlC composites. Mater. Sci. Eng. 2016, 72, 247–256. [CrossRef]
- [64] Bhattacharya, A.K.; Petrovic, J.J. Ductile phase toughening and R-curve behaviour in a B4C-AI cermet. J. Mater. Sci. 1992, 27, 2205–2210. [CrossRef]
9. 著作権:
- 本資料は、「Giovanna Cornacchia, Daniele Dioni, Michela Faccoli, Claudio Gislon, Luigi Solazzi, Andrea Panvini and Silvia Cecchel」による論文です。「Experimental and Numerical Study of an Automotive Component Produced with Innovative Ceramic Core in High Pressure Die Casting (HPDC)」に基づいています。
- 論文出典: https://doi.org/10.3390/met9020217
本資料は上記論文に基づき要約したものであり、商業目的での無断使用を禁じます。
Copyright © 2025 CASTMAN. All rights reserved.