1. 개요:
- 제목: 알루미늄 고압 다이캐스팅의 다이 접착을 유발하는 열역학적 메커니즘 (THERMOMECHANICAL MECHANISMS THAT CAUSE ADHESION OF ALUMINUM HIGH PRESSURE DIE CASTINGS TO THE DIE)
- 저자: 알렉스 먼로 (Alex Monroe)
- 발행 연도: 2021년
- 발행 저널/학회: 미시간 공과대학교, Digital Commons @ Michigan Tech, 박사 학위 논문, 석사 학위 논문 및 보고서 (Michigan Technological University, Digital Commons @ Michigan Tech, Dissertations, Master's Theses and Master's Reports)
- 키워드: HPDC, 접착, 고착, 솔더링, 열역학적, 마찰, 금속간 화합물 (HPDC, adhesion, sticking, soldering, thermomechanical, friction, intermetallic)
2. 연구 배경:
- 연구 주제의 사회적/학문적 맥락: 고압 다이캐스팅(HPDC)은 복잡한 비철 주조품, 특히 자동차 부품의 알루미늄 부품 생산에 널리 사용되는 주조 공정입니다. HPDC의 중요한 문제점 중 하나는 주조재가 다이에 달라붙는 접착 현상으로, 이는 결함으로 이어집니다. 윤활제는 접착을 완화하기 위해 사용되지만, 주조 공극, 기포, 다이 수명 감소 및 기계 마모 증가와 같은 단점을 야기합니다.
- 기존 연구의 한계: 기존 이론은 접착이 알루미늄과 강철 다이 사이의 속도론적으로 제어되는 금속간 화합물 형성 때문이라고 가정합니다. 그러나 이러한 이론에 기반하여 다이 표면을 비반응성 물질로 코팅하는 것은 산업 및 실험실 환경에서 부분적으로만 효과적인 것으로 나타났습니다. 또한 속도론 기반 모델은 심각한 접착이 발생하는 영역을 정확히 예측하지 못하여 추가적인 설명 메커니즘의 필요성을 시사합니다.
- 연구의 필요성: HPDC에서 접착 결함을 예측하고 줄이기 위해서는 속도론적으로 제어되는 금속간 화합물 형성 이론 외에 추가적인 메커니즘을 탐구하고 이해하는 것이 중요합니다. 본 연구는 HPDC에서 고착 및 솔더링에 기여하는 중요한 메커니즘이지만 종종 간과되는 마찰에 초점을 맞추어 연구를 진행합니다.
3. 연구 목적 및 연구 질문:
- 연구 목적: 본 연구는 알루미늄 고압 다이캐스팅(HPDC)에서 접착에 기여하는 열역학적 메커니즘, 특히 마찰에 초점을 맞추어 조사하는 것을 목표로 합니다. 본 연구는 접착이 속도론적으로 제어되는 금속간 화합물 형성에 의해 독점적으로 유발된다는 기존의 이해에 도전하고 마찰 기반 메커니즘을 제안합니다.
- 주요 연구 질문:
- 주조물과 다이 사이의 열역학적 간섭으로 인해 발생하는 마찰은 HPDC에서 고착 및 솔더링에 어느 정도 기여하는가?
- 마찰 기반 모델은 금속간 화합물 형성 속도론에만 기반한 모델에 비해 HPDC에서 접착 현상을 더 정확하게 예측할 수 있는가?
- 연구 가설:
- 솔더링이 주로 속도론적으로 유발되는 상 형성의 결과라면, 실험실 확산 쌍 실험은 산업 솔더링 관찰과 효과적으로 일치하고 예측해야 합니다.
- 마찰이 중요한 요인이라면, 다이 코어의 드래프트 각도를 증가시키면 배출 방향으로의 마찰력 성분 감소로 인해 접촉 면적 감소보다 비례적으로 더 크게 배출력이 감소해야 합니다.
- 솔더링이 마찰과 재료 강도에 영향을 받는다면, 배출 온도를 높이면 합금의 강도가 감소하여 마찰 전단 응력에 대한 저항력이 약해지므로 솔더링 가능성이 증가해야 합니다.
4. 연구 방법론
- 연구 설계: 본 연구는 HPDC에서 접착 메커니즘을 분석하기 위해 실험적 조사와 전산 모델링을 결합한 혼합 방법론적 접근 방식을 채택했습니다. 여기에는 다음이 포함됩니다.
- 배출력을 측정하고 솔더링 현상을 관찰하기 위한 상온 및 고온 배출 테스트를 포함한 실험 연구.
- 주조물-다이 계면에서 응고, 냉각, 열 응력 및 마찰을 시뮬레이션하기 위한 1차원(1D) 및 3차원(3D) 수치 모델링.
- 데이터 수집 방법: 데이터는 다음을 통해 수집되었습니다.
- 상온 배출 테스트를 위해 오메가다인 로드 셀이 장착된 티니우스 및 올슨 인장 시험기를 사용하여 배출력 측정.
- 고온 배출 테스트를 위해 k형 열전대로 온도를 기록하고 로드 셀로 배출력을 측정. 변위는 LVDT로 측정.
- 산업 주조물의 3D 모델링을 위해 MAGMASOFT에서 생성된 시뮬레이션 데이터와 1D 열역학적 모델링을 위한 MATLAB 데이터.
- 분석 방법: 수집된 데이터는 다음을 사용하여 분석되었습니다.
- 배출력 및 솔더링 가능성을 예측하기 위한 쿨롱 및 트레카 마찰 모델 적용.
- 다양한 합금이 배출력에 미치는 영향을 비교하기 위한 쌍체 스튜던트 t-검정을 포함한 통계 분석.
- 계면 강도, 접촉 압력 및 마찰 계수와 같은 매개변수를 결정하기 위해 엑셀 솔버를 사용하여 실험 데이터를 마찰 모델에 피팅.
- 마찰 기반 접근 방식의 유효성을 검증하기 위해 모델 예측과 실험 관찰 및 산업 사례 연구 비교.
- 연구 대상 및 범위: 연구는 다음 사항에 초점을 맞추었습니다.
- 축 대칭을 가정하여 모델링 및 실험을 단순화하기 위해 관형 주조물 내의 원통형 다이(코어) 형상.
- 알루미늄 합금: 주로 A380 및 A356, 합금 조성 효과를 평가하기 위해 A362, A365 및 A518에 대한 추가 시뮬레이션. 화학 조성은 표 4에 자세히 설명되어 있습니다.
- 다이 재료: H13 강철, H13 High K 강철 및 인코넬이 시뮬레이션에서 고려되었습니다(속성은 표 5에 있음).
- 조사된 변수: 드래프트 각도(0.5°~25°), 배출 온도(상온~555°C), 합금 조성, 다이 재료, 주조 두께 및 코어 직경.
5. 주요 연구 결과:
- 주요 연구 결과:
- 주요 메커니즘으로서의 마찰: 상온 배출 테스트 결과, 배출력은 본질적인 접착력을 설명하기 위한 보정 항 없이 마찰 모델에 의해 잘 예측되는 간섭 끼워맞춤과 유사하게 거동하는 것으로 나타났습니다. "최적의 피팅은 계면 강도가 0으로 설정되고, 접촉 압력이 5.9 MPa, 마찰 계수가 0.59일 때 달성됩니다. 최적의 피팅은 “마찰 모델” 선으로 플롯되었으며, 이 접근 방식은 계면 강도만의 접착을 가정하여 플롯되었습니다. 이것은 점선 “접착 모델” 선이며, 데이터에 대한 피팅이 좋지 않습니다." (그림 20).
- 드래프트 각도 효과: 드래프트 각도를 증가시키면 마찰 모델 예측과 일치하게 배출력이 크게 감소했습니다. "드래프트 각도 증가에 따라 배출력이 감소하는 경향을 보이지만 합금에 미치는 영향은 미미한 모든 배출력 측정값을 플롯한 것"이 그림 18에 나와 있습니다.
- 고온 배출 및 솔더링: 고온 배출 테스트를 통해 고온에서 솔더링이 발생하는 것을 확인했습니다. "가설은 고온 배출 테스트에 의해 부분적으로 확인되었는데, 단일 주조 사이클 내에서 알루미늄이 코어에 접착되는 것으로 나타났습니다(그림 30)."
- 3D 시뮬레이션 검증: 트레카 마찰 계수를 사용한 3D 시뮬레이션은 산업 다이캐스팅 사례에서 솔더링 위치를 성공적으로 예측하여 생산 툴링에서 관찰된 솔더링 패턴과 일치했습니다. "솔더링 위치와 상대적 심각도는 이 마찰 기반 예측을 사용하여 잘 예측됩니다." (그림 35).
- 코팅은 마찰 감소: AlCrN 코팅은 마찰 계수를 감소시켜 코팅이 마찰을 줄임으로써 고착을 완화하는 주요 원인임을 시사합니다. "코팅은 마찰 계수를 0.59에서 0.5로 18% 감소시켰습니다. 이는 코팅이 고착을 줄이는 주요 이유가 마찰을 줄이는 것이라는 것을 시사합니다."
- 통계적/정성적 분석 결과:
- 상온에서 합금의 무의미성: 쌍체 스튜던트 t-검정 결과, 상온에서 합금 362와 380 사이의 배출력에 유의미한 차이가 없는 것으로 나타났습니다. "두 합금의 데이터에 대해 쌍체 스튜던트 t-검정을 수행했으며, 쌍체 테스트에 대한 t-통계량은 0.94로 계산되었습니다. 이는 95% 유의 수준을 가정할 때 임계값 1.71보다 작습니다. 이는 362와 380의 배출력이 다르지 않다는 것을 의미합니다…"
- 마찰 모델 피팅: 마찰 모델은 접착 전용 모델에 비해 실험 데이터에 더 나은 피팅을 제공했습니다. "최적의 피팅은 “마찰 모델”로 달성되며, 접착 항을 추가해도 개선되지 않습니다. 접착 전용 모델은 데이터와 일치하지 않습니다." (그림 20).
- 데이터 해석: 결과는 열역학적 간섭으로 인해 발생하는 마찰이 HPDC에서 고착 및 솔더링 현상을 포함한 알루미늄 접착을 유발하는 주요 메커니즘이라는 가설을 강력하게 뒷받침합니다. 실험 및 시뮬레이션 결과는 HPDC에서 접착이 속도론 기반 금속간 화합물 형성 이론만을 고려하는 것보다 마찰 모델에 의해 더 잘 설명된다는 것을 나타냅니다. 접촉 압력과 배출 온도는 접착에 영향을 미치는 중요한 요인으로 확인되었습니다.
- 그림 목록:
- Figure 1: The moving half of a typical die casting die [2].
- Figure 2: (a) An example of sticking. Both images courtesy of Mercury Marine. (b) An example of soldering on a die insert.
- Figure 3: Temperature dependence of the proposed dimensionless ejection force, Fej * and ejection shear stress, Tr.
- Figure 4: (a) Conceptual plot of the Coulomb model where the ejection stress is low relative to the part strength at low temperatures, and the part sticks to the die. (b) Conceptual plot at high temperature where all ejection stresses are large relative to the part strength, and the part fails. Figure adapted from Widerøe and Welo [15].
- Figure 5: Stages of soldering as proposed by Chu et al. in 1993 [9].
- Figure 6: Soldering that occurs on a die that has a non-reactive coating identified by Wang et al. [8]. Lubricant spray was reduced, but the soldering (red circles) and sticking still occur (Image courtesy of Mercury Marine).
- Figure 7: Conceptual casting for developing a thermomechanical theory of soldering and sticking. Radii smaller than ri are the die steel core, and radii greater than ro are the die steel mold.
- Figure 8: Schematic of the common thermodynamics and kinetics-based theory of soldering and sticking.
- Figure 9: The predicted time to grow a 0.1 mm thick intermetallic layer [78]–[80], [83], [84]. A single activation energy of 190 kJ/mol provides good agreement with measured reaction rates both above and below the melting temperature of 660°C
- Figure 10: (a) Illustration of a typical aluminum iron diffusion couple [78]. (b) One dimensional intermetallic growth rate when the liquid is either initially pure or saturated with iron [85].
- Figure 11: (a) Static steel cylinder (12.7 mm diameter) dip tests in various aluminum alloys showed that pure aluminum dissolves steel more slowly than commercial casting alloys [89]. (b) Static steel cylinder (12.7 mm diameter) dip tests showed that the structural alloy Silafont 36 (A367) dissolves steel slower than the more common A390 diecasting alloy [88].
- Figure 12: Illustration of joints between steel and aluminum under the shear of the ejection force, Fe without (a,b) and with an intermetallic layer (c,d). When the bond strength is low a clean separation at the aluminum/steel interface is normally observed (a) Casting failure may occur due to the low strength of aluminum at elevated temperature. (b). Roll bonded, brazed, and friction stir welded joints typically fracture within the intermetallic layer when it is present (c), while HPDC conditions often contain an intermetallic layer with the fracture in die casting (d).
- Figure 13: A simplified schematic based on Figure 3 where only the sticking factor is considered.
- Figure 14: Conceptual model of the casting/die interface where Fej is the ejection force and Ac is the total contact area. tAl, tej, and tFe are the shear strength of the casting, interface, and die, respectively.
- Figure 15: Different factors that affect the local contact area in the die casting process.
- Figure 16: Schematic of room temperature ejection mold.
- Figure 17: Image of the fixture for measuring the ejection force. The top and bottom of the fixture could pivot by a few degrees to correct for misalignment.
- Figure 18: A plot of all ejection force measurements showing a trend of decreasing ejection force with increasing draft angle but little effect of alloy.
- Figure 19: Schematic of core denoting the contact area used to fit (eq. 6) to the experimental data (Figure 18).
- Figure 20: The best fit is achieved with the “Friction Model” and is not improved by adding the adhesion term. The adhesion only model does not match the data.
- Figure 21: Image of all 7 cores after over 10 casting cycles showing no buildup from the cast aluminum.
- Figure 22: Comparison of AlCrN coated cores showing no difference between the smooth (Diamond Polished) and rough (220 Grit Stoned) surface preparation.
- Figure 23: Friction model fit to the AlCrN coated cores versus the uncoated cores. The 95% confidence interval based on a minimum of 6 experiments for each data point is shown.
- Figure 24: HPDC soldering occurs when the Tresca friction factor is larger than a critical value, and it decreases with lower ejection temperatures, (subset of Figure 3).
- Figure 25: The L-H relationship as proposed in eqs. 9 through 12. UTS was calculated assuming the onset of necking where N(T)/1 – М(Т).
- Figure 26: Bulk stress state prior to ejection due to the thermal interference between the casting and core.
- Figure 27: Thermal strain compared to the yield strain as calculated using an 0.002 offset of elastic modulus in eq. 18.
- Figure 28: Predicted soldering likelihood of a cylindrical casting (eq. 21) based on the alloy described in eq. 9 versus the ejection temperature.
- Figure 29: General assembly view of the hot ejection test apparatus the casting, core, sand mold, and support plate.
- Figure 30: (a) Example of experimental rig prepared for casting. (b) Incipient soldering clearly shown on the case where ejection occurred at 550°C.
- Figure 31: Casting surface for hot ejection test showing surface shrinkage (casting) and aluminum buildup (core).
- Figure 32: Rectangular example showing that the contact pressure is proportional to the thickness of the casting relative to the bearing area, Ac, on the core.
- Figure 33: Example die simulated in MAGMASOFT to test the validity of the Tresca friction factor to predict HPDC soldering.
- Figure 34: Second industrial HPDC example for comparison of the Tresca friction factor with HPDC soldering.
- Figure 35: Comparison of the contact pressure scaled by UTS in MAGMA (a) and (c) with the production die casting die at the end of a production run (b) and (d).
- Figure 36: Predicted soldering friction factor (right hand axis) vs simulation time. The temperatures at the same locations are plotted on the left-hand axis. Both locations exhibit increasing then decreasing soldering tendency related to the local temperature of the casting.
- Figure 37: Strength vs test temperature of typical wrought aluminum alloys in the as cast state [97]. UTS was assumed to occur at the onset of necking.
- Figure 38: The limits of ejection to avoid sticking and soldering. This is the axis-symmetric case of the general conceptual model proposed in the introduction (Figure 3).
- Figure 39: Density (a) and enthalpy (b) of the alloys in Table 4 as predicted by ThermoCalc equilibrium calculations [75]. Cumulative heat release (c) during solidification and cooling of the alloys. (d) Linearized thermal conductivity vs temperature for both alloys based on available literature measurements [100], [101].
- Figure 40: (a) Average casting temperature for a 12 mm tube casting with a 100 mm inner diameter. (b) Modeled contact pressure vs ejection time.
- Figure 41: A schematic that illustrates the limits on acceptable ejection times.
- Figure 42: Predicted average casting temperature (a) and ejection force (b) of the alloys from Table 4.
- Figure 43: Main effects plots for prediction of the range of possible ejection times for a core in a tubular casting of various, thickness, casting alloy, core diameter, initial die temperature.
- Figure 44: The main effect if die materials for the full factorial simulation. Different die materials are discerned by their thermal conductivity in Table 5.
- Figure 45: Enthalpy release of alloys in on a per volume basis during solidification and cooling.
- Figure 46: Soldering severity ranking from the NADCA Product Design Standards indicating A518 is castable but it has the worst die filling capacity and anti-solder capability [103].
- Figure 47: Cumulative heat release during solidification for A380 with 0.2 wt% iron and 0.2 wt% manganese compared to the same alloy with 1 wt% iron and 0.3 wt% manganese. There is a 3% reduction in heat released when iron and manganese are lowered.
![Figure 1: The moving half of a typical die casting die [2].](https://castman.co.kr/wp-content/uploads/image-145-1024x726.webp)
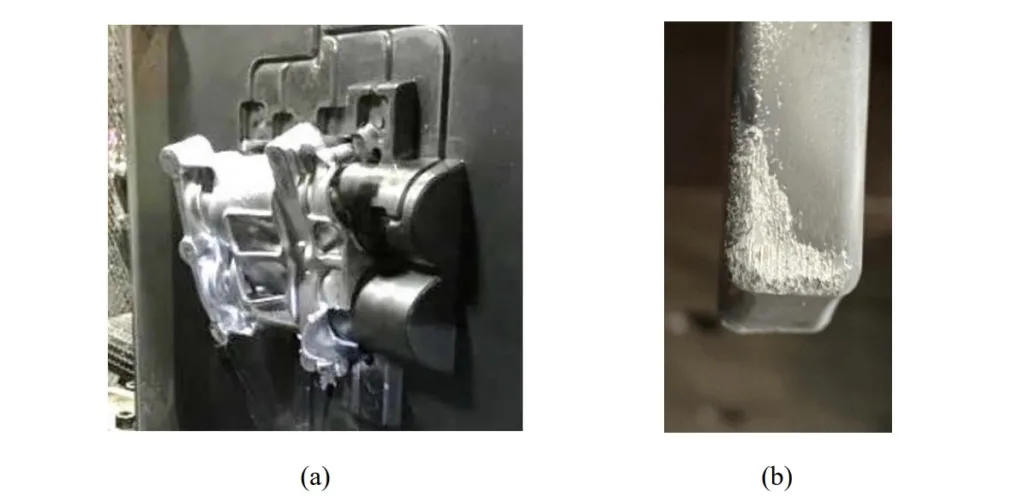
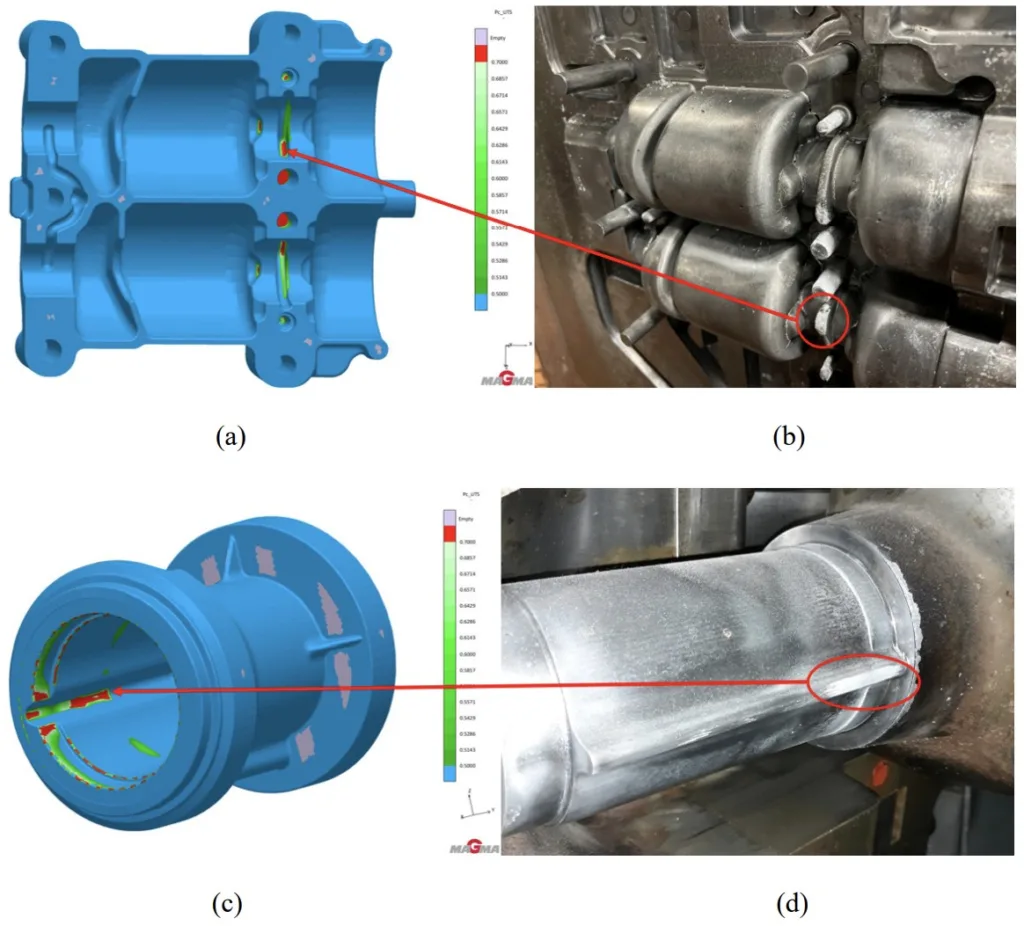
6. 결론 및 논의:
- 주요 결과 요약: 본 연구는 주조물과 다이 사이의 열역학적 간섭으로 인한 마찰이 HPDC 다이에 알루미늄이 접착되는 주요 메커니즘이며, 고착 및 솔더링 현상을 모두 포괄한다는 결론을 내렸습니다. 상온 배출 테스트 결과, 본질적인 접착력 항이 필요 없이 배출력이 마찰과 유사하게 거동하는 것으로 나타났습니다. 고온 배출 테스트 및 3D 시뮬레이션은 솔더링이 높은 트레카 마찰 계수와 관련이 있음을 보여주어 마찰의 역할을 더욱 검증했습니다. 접촉 압력을 최소화하는 것이 HPDC에서 접착 문제를 줄이는 가장 효과적인 전략으로 확인되었습니다.
- 연구의 학문적 의의: 본 연구는 종종 간과되었던 마찰 메커니즘의 중요성을 강조함으로써 HPDC에서 접착에 대한 이해에 중요한 변화를 제공합니다. 본 연구는 접착을 예측하고 완화하기 위한 새로운 열역학적 프레임워크를 도입하여 관찰된 현상에 대한 보다 포괄적인 설명을 제공합니다.
- 실용적 의미: 본 연구는 접착을 줄이기 위해 주조물 배출 온도를 최대화하고 접촉 압력을 최소화하는 데 초점을 맞춘 HPDC 공정에 대한 최적 설계 방법론을 제공합니다. 트레카 마찰 계수를 통합한 3D 시뮬레이션 도구를 사용하여 솔더링 위치를 예측하고 다이 및 공정 설계를 최적화하는 것이 좋습니다. 합금 선택 및 공정 매개변수 조정은 마찰 모델을 기반으로 접착을 최소화하고 주조 품질을 향상시키도록 안내할 수 있습니다.
- 연구의 한계: 고온 배출 테스트는 모델에서 가정한 이상적인 축 대칭 케이스에서 벗어나 균일한 접촉을 달성하는 데 어려움이 있었습니다. 고온 배출 실험에서 테스트된 온도 범위는 변형 경화 계수의 변화가 제한적이어서 해당 특정 테스트에서 솔더링 예측의 민감도에 영향을 미칠 수 있습니다.
7. 향후 후속 연구:
- 후속 연구 방향: 향후 연구에서는 윤활제 및 코팅과 코어의 표면 마감 사이의 상호 작용을 조사하여 접착 제어 전략을 더욱 개선해야 합니다. Al-Si 합금의 고온 기계적 특성, 특히 철 및 망간 함량 변화에 대한 추가 연구가 재료 기반 접착 감소 솔루션을 강화하기 위해 필요합니다. 마찰을 줄이는 것을 목표로 하는 다이 코팅의 지속적인 개발 및 테스트도 중요합니다.
- 추가 탐구가 필요한 영역: 마찰 전단 응력에 저항하기 위해 고온 강도를 향상시키는 데 초점을 맞춘 합금 최적화 전략은 추가 조사가 필요한 영역입니다. 열 전달을 관리하고 까다로운 주조 합금에서 고착 경향을 줄이기 위해 낮은 열전도율 다이 재료를 활용할 가능성을 더 탐구해야 합니다.
8. 참고 문헌:
- [1] S. P. Udvardy, “2019 State of the Die Casting Industry,” Die Casting Engineer, no. January, p. 6, 2019.
- [2] 2018 Product Specification Standards for Die Castings, 2018th ed. North American Die Casting Association.
- [3] R. J. Donahue and G. K. Sigworth, “Die Casting Alloys that will Allow the Die Caster to Compete with Alloys A356, A357, 358 and 359 in PM Applications,” p. 17.
- [4] Aoyama, Shunzo, Akase, Makota, Sakamoto, Katsumi, and Umemura, Teruyoshi, “Die Lubricant Deposit and Its Effect on Ejection in Die Casting,” vol. T91-112.
- [5] R. A. Miller, “Fluidity from a Heat Transfer Perspective,” in NADCA Transactions, Ohio, Sep. 2016, vol. T16-081. Accessed: Apr. 10, 2018. [Online]. Available: https://www.diecasting.org/archive/transactions/T16-081.pdf
- [6] R. N. Lumley, I. J. Polmear, H. Groot, and J. Ferrier, “Thermal characteristics of heat-treated aluminum high-pressure die-castings,” Scr. Mater., vol. 58, no. 11, pp. 1006–1009, Jun. 2008, doi: 10.1016/j.scriptamat.2008.01.031.
- [7] D. Blondheim, Jr. and A. Monroe, “Macro Porosity Formation - A Study in High Pressure Die Casting,” Int. J. Met., 2021, doi: https://doi.org/10.1007/s40962-021-00602-x.
- [8] B. Wang, G. R. Bourne, A. L. Korenyi-Both, S. P. Midson, and M. J. Kaufman, “An investigation of the use of PVD die coatings to minimize or eliminate lubrication during high pressure die casting,” in North American Die Casting Congress, 2016, vol. T16-061.
- [9] Chu, Yeou-Li, Cheng, Patrick, and Shivpuri, Rajiv, “Soldering Phenomenon in Aluminum Die Casting: Possible Causes and Cures,” in North American Die Casting Congress, Cleveland, OH, Oct. 1993, pp. 361–372.
- [10] Tosa, Hiroki and Urakami, Akio, “Factors Influencing Die Casting Ejection," in North American Die Casting Congress, Chicago, IL, 1972, vol. T72-041.
- [11] B. Wang, G. R. Bourne, A. L. Korenyi-Both, A. K. Monroe, S. P. Midson, and M. J. Kaufman, “Method to evaluate the adhesion behavior of aluminum-based alloys on various materials and coatings for lube-free die casting,” J. Mater. Process. Technol., vol. 237, pp. 386–393, 2016, doi: 10.1016/j.jmatprotec.2016.06.031.
- [12] S.-B. Kim, Y.-H. Yim, and D.-H. Roh, “Prediction of Die Soldering in Die Casting by a Numerical Algorithm based on the Combination of Thermal and Fluid Factors Verified with Experimental Results,” in Die Casting Congress and Tabletop, Cleveland, OH, 2019, p. 9.
- [13] K. Domkin, J. H. Hattel, and J. Thorborg, “Modeling of high temperature- and diffusion-controlled die soldering in aluminum high pressure die casting,” J. Mater. Process. Technol., vol. 209, no. 8, pp. 4051–4061, 2009, doi: 10.1016/j.jmatprotec.2008.09.031.
- [14] D. Tabor, “Friction - the present state of our understanding,” J. Lubr. Technol., vol. 103, no. 2, pp. 169–179, Apr. 1981.
- [15] F. Widerøe and T. Welo, “Conditions for Sticking Friction between Aluminium Alloy AA6060 and Tool Steel in Hot Forming,” Key Eng. Mater., vol. 491, pp. 121-128, Sep. 2011, doi: 10.4028/www.scientific.net/KEM.491.121.
- [16] H. Wang and C. A. Loong, “Experimental Studies on Ejection FORCES of a die cast Aluminum Part,” in North American Die Casting Congress, Cleveland, OH, 1999, vol. T99-052.
- [17] M. J. Roy, D. M. Maijer, and L. Dancoine, “Constitutive behavior of as-cast A356," Mater. Sci. Eng. A, vol. 548, pp. 195–205, Jun. 2012, doi: 10.1016/j.msea.2012.03.106.
- [18] Shankar, Samantha and Apelian, Diran, “Die Soldering: Mechanism of the interface reaction between molten aluminum alloy and tool steel,” vol. 33B, no. June 2002, pp. 465–476, doi: 10.1007/s11663-002-0057-7.
- [19] ASM Handbook: Welding, Brazing, and Soldering, vol. 6. ASM International (R), Materials Park, Ohio, USA, 1993.
- [20] A. Long, D. Thornhill, C. Armstrong, and D. Watson, “Predicting die life from die temperature for high pressure dies casting aluminium alloy,” Appl. Therm. Eng., vol. 44, pp. 100–107, Nov. 2012, doi: 10.1016/j.applthermaleng.2012.03.045.
- [21] A. Long, D. Thornhill, C. Armstrong, and D. Watson, “Determination of the heat transfer coefficient at the metal–die interface for high pressure die cast AlSi9Cu3Fe," Appl. Therm. Eng., vol. 31, no. 17–18, pp. 3996–4006, Dec. 2011, doi: 10.1016/j.applthermaleng.2011.07.052.
- [22] Z. W. Chen, “Formation and progression of die soldering during high pressure die casting,” Mater. Sci. Eng. A, vol. 397, no. 1–2, pp. 356–369, 2005, doi: 10.1016/j.msea.2005.02.057.
- [23] Y. Zhu, D. Schwam, J. F. Wallace, and S. Birceanu, “Evaluation of soldering, washout and thermal fatigue resistance of advanced metal materials for aluminum die-casting dies,” Mater. Sci. Eng. A, vol. 379, no. 1–2, pp. 420–431, 2004, doi: 10.1016/j.msea.2004.03.020.
- [24] Chu, Yeou-Li, Balasubramaniam, Srikanth, Rajan, Rajendra, and Shivpuri, Rajiv, "A Study of the Cast Alloy-Die Surface Interactions in Aluminum Die Casting,” in North American Die Casting Congress, Minneapolis, MN, 1997, vol. T97-075. [Online]. Available: https://diecasting.org/wcm/Technology/Technical_Archive/wcm/Technology/Technical_Archive.aspx?hkey=62f825ba-424c-41c3-b10f-3b860771ffa4
- [25] S. Gulizia, M. Z. Jahedi, and E. D. Doyle, “Performance evaluation of PVD coatings for high pressure die casting,” Surf. Coat. Technol., vol. 140, no. 3, pp. 200-205, Jun. 2001, doi: 10.1016/S0257-8972(01)01037-4.
- [26] Hairy, P. and Richard, M., “Reduction of Sticking in Pressure Die Casting by Surface Treatment,” in North American Die Casting Congress, 1997, vol. T97-102.
- [27] K. Bobzin, T. Brögelmann, U. Hartmann, and N. C. Kruppe, "Analysis of CrN/AlN/Al2O3 and two industrially used coatings deposited on die casting cores after application in an aluminum die casting machine,” Surf. Coat. Technol., vol. 308, pp. 374-382, Dec. 2016, doi: 10.1016/j.surfcoat.2016.09.040.
- [28] Q. Han and S. Viswanathan, “Analysis of the Mechanism of Die Soldering in Aluminum Die Casting,” Metall. Mater. Trans. A, vol. 34A, no. January, pp. 139–146, 2003.
- [29] Shankar, Sumanth and Apelian, Diran, “Mechanism and Preventive Measures for Die Soldering during Al Casting in a Ferrous Mold,” JOM, vol. 54, no. August 2002, pp. 47–54, doi: 10.1007/BF02711867.
- [30] C. Mitterer, F. Holler, F. Üstel, and D. Heim, "Application of hard coatings in aluminium die casting – soldering, erosion and thermal fatigue behaviour,” Surf. Coat. Technol., vol. 125, no. 1–3, pp. 233–239, Mar. 2000, doi: 10.1016/S0257-8972(99)00557-5.
- [31] Norstrom, Lars-Ake, Klarenfjord, Bengt, and Svensson, Maths, “General Aspects on 'Washout' Mechanisms in Aluminum Die Casting Dies," 1993, vol. T93-075–1.
- [32] M. Yu, R. Shivpuri, and R. A. Rapp, “Effects of Molten Aluminum on H13 Dies and Coatings,” J. Mater. Eng. Perform., vol. 4, no. 2, pp. 175–181, Apr. 1995.
- [33] R. J. Barnhurst, D. Argo, and W. Walkington, “NADCA Sponsored Research: The Causes of Soldering in Zinc Die Casting,” in Die Casting Congress, 1997, vol. T97-033.
- [34] M. Stern, “PROGRESS_OF_DIE-CASTING_INDUSTRY,” in Transactions of the American Foundrymen's Association, 1930, vol. 37, pp. 723–733.
- [35] W. Bonsack, “Trends in Aluminum Casting Alloys,” in Transactions of the American Foundrymen's Association, 1950, vol. 76, pp. 69–75.
- [36] D. L. Colwell and E. Trela, “New Aluminum Die Casting Alloys,” in Transactions of the American Foundrymen's Association, 1954, vol. 93, pp. 359–364.
- [37] D. L. Colwell and O. Tichy, “Machinability of Aluminum Die Castings,” in Transactions of the American Foundrymen's Association, 1956, vol. 92, pp. 236–241.
- [38] S. U. Siena, “Die Casting Aluminum Alloys by the Cold Chamber Process,” 1944.
- [39] W. Bonsack, “Iron-The Problematic Factor in Quality Of Aluminum Alloy Die Castings," in Transactions of the American Foundrymen's Association, 1961, vol. 114, pp. 712-720.
- [40] Holz, Earl K., “Trouble Shooting Aluminum Die Casting Quality Problems,” in 7th SDCE International Die Casting Congress, Chicago, IL, 1972, vol. T72-043.
- [41] Kajoch, Wladyslaw and Fajkiel, Alexander, “Testing the Soldering Tendencies of Aluminum Die Casting Alloy,” in North American Die Casting Congress, Detroit, MI, Oct. 1991, vol. T91-034, pp. 67–74.
- [42] W. Jonsson, “THE EFFECT OF IRON AND MAGNESIUM ON TEH PROPERTIES OF ALUMINUM DIE CASTING ALLOYS,” Nov. 1964, p. 8.
- [43] Chu, Yeou-Li, Cheng, Patrick, and Shivpuri, Rajiv, “Investigation of the soldering problem in aluminum die casting - Phenomena, mechanism and protection,” National Science Foundation Engineering Research Center for Net Shape Manufacturing, NADCA Technical Archive, ERC/NSM-C-93-36, Sep. 1993.
- [44] Y. Tsuchiya, H. Kawaura, K. Hashimoto, H. Inagaki, and T. Arai, “Core Pin Failure in Aluminum Die Casting and the Effect of Surface Treatment,” in The Many Faces of Die Casting, Minneapolis, MN, 1997, vol. T97-103.
- [45] Z. W. Chen, D. T. Fraser, and M. Z. Jahedi, “Structures of intermetallic phases formed during immersion of H13 tool steel in an Al–11Si–3Cu die casting alloy melt," Mater. Sci. Eng. A, vol. 260, no. 1–2, pp. 188–196, Feb. 1999, doi: 10.1016/S0921-5093(98)00963-0.
- [46] R. J. Barnhurst, D. Argo, and W. Walkington, “NADCA Sponsored Research: The Causes of Soldering in Zinc Die Casting,” in Die Casting Congress, 1997, vol. T97-033.
- [47] J. Song, X. Wang, T. DenOuden, and Q. Han, “Evolution of Intermetallic Phases in Soldering of the Die Casting of Aluminum Alloys,” Metall. Mater. Trans. A, vol. 47, no. 6, pp. 2609–2615, 2016, doi: 10.1007/s11661-016-3454-2.
- [48] A. Duarte, F. J. Oliveira, and F. M. Costa, “Characterisation of interface formed at 650°C between AISI H13 steel and Al–12Si–1Cu aluminium melt," Int. J. Cast Met. Res., vol. 23, no. 4, pp. 231–239, Aug. 2010, doi: 10.1179/136404610X12665088537455.
- [49] S. Sumanth and A. Diran, “The Role of Aluminum Alloy Chemistry and Die Material on Die Soldering,” in North American Die Casting Congress, Cleveland, OH, 1999, vol. T99-083. [Online]. Available: https://diecasting.org/wcm/Technology/Technical_Archive/wcm/Technology/Technical_Archive.aspx?hkey=62f825ba-424c-41c3-b10f-3b860771ffa4
- [50] M. Vilaseca, S. Molas, and D. Casellas, “High temperature tribological behaviour of tool steels during sliding against aluminium,” Wear, 2011, doi: 10.1016/j.wear.2011.07.007.
- [51] P. Terek et al., “Effects of die core treatments and surface finishes on the sticking and galling tendency of Al-Si alloy casting during ejection,” Wear, vol. 356–357, pp. 122-134, Jun. 2016, doi: https://doi.org/10.1016/j.wear.2016.03.016.
- [52] H. Cagin, “Techniques for Removing Stuck Castings," in NADCA Transactions, Jun. 1981, vol. T81-025, p. 4.
- [53] P. Terek et al., “The Influence of CrAlN Coating Chemical Composition on Soldering Resistance in Contact with Al-Si-Cu Alloy,” Mater. Proc., vol. 2, no. 1, p. 28, May 2020, doi: 10.3390/CIWC2020-06837.
- [54] P. Terek, L. Kovačević, A. Miletić, D. Kukuruzović, S. Baloš, and B. Škorić, "Improved ejection test for evaluation of soldering tendency of cast alloy to die core materials,” J. Mater. Process. Technol., vol. 266, pp. 114–124, Apr. 2019, doi: 10.1016/j.jmatprotec.2018.10.038.
- [55] Chen, Z. W. and Jahedi, M. Z., “Die erosion and its effect on soldering formation in high pressure die casting of aluminum alloys,” Mater. Des., vol. 20, pp. 303-309, 1999.
- [56] J. Jerina and M. Kalin, “Initiation and evolution of the aluminium-alloy transfer on hot-work tool steel at temperatures from 20 °C to 500 °C,” Wear, vol. 319, no. 1–2, pp. 234-244, Nov. 2014, doi: 10.1016/j.wear.2014.07.021.
- [57] R. Kimura, M. Yoshida, G. Sasaki, J. Pan, and H. Fukunaga, “Characterization of heat insulating and lubricating ability of powder lubricants for clean and high quality die casting,” J. Mater. Process. Technol., vol. 130–131, pp. 289–293, Dec. 2002, doi: 10.1016/S0924-0136(02)00808-7.
- [58] N. Nishi, “Challenge to Ultra Thin-Wall Zinc Die Casting under 0.5 mm Thickness," Cast. Eng., no. September 2007, p. 5, 2007.
- [59] MAGMAsoft. Kackerstrasse 11, 52072 Aachen, Germany: MAGMA Gmbh, 2019.
- [60] T. Cedorge and J. Colton, “Draft angle and surface roughness effects on sterolighography molds," Polym. Eng. Sci. Newtown, vol. 40, no. 7, p. 1581, Jul. 2000.
- [61] A. E. Palmer and J. S. Colton, “Failure mechanisms in stereolithography injection molding tooling,” Polym. Eng. Sci., vol. 40, no. 6, pp. 1395–1404, Jun. 2000, doi: 10.1002/pen.11269.
- [62] G. T. Pham and J. S. Colton, “Ejection force modeling for stereolithography injection molding tools,” Polym. Eng. Sci., vol. 42, no. 4, pp. 681–693, Apr. 2002, doi: 10.1002/pen.10981.
- [63] B. Omar and K. Barney, “Prediction of Local Part-Mold and Ejection Force in Injection Molding,” Trans. ASME, vol. 127, no. August 2005, pp. 598–604, Aug. 2005, doi: 10.1115/1.1951785.
- [64] S. Tetsuo, K. Nobuhiro, S. Kenji, K. Yoshikazu, and T. Ayumu, “An experimental study on ejection forces of injection molding,” J. Int. Soc. Precis. Eng. Nanotechnol., vol. 24, pp. 270–273, 2000, doi: 0141-6359/00/.
- [65] M. Sorgato, D. Masato, and G. Lucchetta, “Effects of machined cavity texture on ejection force in micro injection molding,” Precis. Eng., vol. 50, pp. 440–448, 2017, doi: 10.1016/j.precisioneng.2017.06.019.
- [66] Monroe, Alexander, Gaddam, Deepika, and Glim, Beau, “Preliminary Study on Ejection Force Prediction,” NADCA Congr. Tabletop, vol. T16-083, 2016.
- [67] A. L. ALTIERI and P. H. STEEN, “Adhesion Upon Solidification and Detachment in the Melt Spinning of Metals,” Metall. Mater. Trans. B, vol. VOLUME 45B, pp. 2262-2268, Dec. 2014, doi: 10.1007/s11663-014-0128-6.
- [68] M. D. Hanna, “Tribological evaluation of aluminum and magnesium sheet forming at high temperatures,” Wear, vol. 267, no. 5–8, pp. 1046–1050, Jun. 2009, doi: 10.1016/j.wear.2009.01.007.
- [69] H. Kitano, K. Dohda, M. Kalin, and K. F. Ehmann, “Galling growth analysis in metal forming,” Manuf. Lett., vol. 16, pp. 32–35, Apr. 2018, doi: 10.1016/j.mfglet.2018.03.003.
- [70] E. Huttunen-Saarivirta, L. Kilpi, T. J. Hakala, J. Metsäjoki, and H. Ronkainen, "Insights into the behaviour of tool steel-aluminium alloy tribopair at different temperatures,” Tribol. Int., vol. 119, pp. 567–584, Mar. 2018, doi: 10.1016/j.triboint.2017.11.041.
- [71] L. Wang and H. Yang, “Friction in aluminium extrusion—part 2: A review of friction models for aluminium extrusion,” Tribol. Int., vol. 56, pp. 99–106, Dec. 2012, doi: 10.1016/j.triboint.2012.06.006.
- [72] X. Ma, M. de Rooij, and D. Schipper, “A load dependent friction model for fully plastic contact conditions,” Wear, vol. 269, no. 11–12, pp. 790–796, Oct. 2010, doi: 10.1016/j.wear.2010.08.005.
- [73] X. Ma, M. B. de Rooij, and D. J. Schipper, “Friction conditions in the bearing area of an aluminium extrusion process,” Wear, vol. 278–279, pp. 1–8, Mar. 2012, doi: 10.1016/j.wear.2011.11.001.
- [74] L. Wang, J. Zhou, J. Duszczyk, and L. Katgerman, “Friction in aluminium extrusion-Part 1: A review of friction testing techniques for aluminium
- [75] J. Shigley, C. Mischke, and R. Budynas, Mechanical Engineering Design, 7th Edition. McGraw-Hill, 2004.
- [76] MATLAB. Natick, Massachusetts: Mathworks, 2020.
- [77] J. O. Andersson, T. Helander, P. F. Shi, and B. Sundman, Thermo-Calc and DICTRA, Computational tools for material science. 2002.
- [78] H. Springer, A. Kostka, J. F. dos Santos, and D. Raabe, “Influence of intermetallic phases and Kirkendall-porosity on the mechanical properties of joints between steel and aluminium alloys,” Mater. Sci. Eng. A, vol. 528, no. 13–14, pp. 4630–4642, May 2011, doi: 10.1016/j.msea.2011.02.057.
- [79] H. Springer, A. Kostka, E. J. Payton, D. Raabe, A. Kaysser-Pyzalla, and G. Eggeler, “On the formation and growth of intermetallic phases during interdiffusion between low-carbon steel and aluminum alloys,” Acta Mater., vol. 59, no. 4, pp. 1586–1600, Feb. 2011, doi: 10.1016/j.actamat.2010.11.023.
- [80] V. Jindal, V. C. Srivastava, A. Das, and R. N. Ghosh, “Reactive diffusion in the roll bonded iron-aluminum system,” Mater. Lett., vol. 60, no. 13–14, pp. 1758–1761, Jun. 2006, doi: 10.1016/j.matlet.2005.12.013.
- [81] Z. W. Chen, “Formation and progression of die soldering during high pressure die casting,” Mater. Sci. Eng. A, vol. 397, no. 1–2, pp. 356–369, 2005, doi: 10.1016/j.msea.2005.02.057.
- [82] C. Z. W., J. M.Z., and L. J.A., “Metallurgical Phenomena in Die/Casting Interfacial Regions During High Pressure Die Casting of Aluminum Alloys,” in North American Die Casting Congress, Cleveland, OH, 1999, vol. T99-084.
- [83] A. Bouayad, C. Gerometta, A. Belkebir, and A. Ambari, “Kinetic interactions between solid iron and molten aluminium,” Mater. Sci. Eng. A, vol. 363, no. 1–2, pp. 53-61, Dec. 2003, doi: 10.1016/S0921-5093(03)00469-6.
- [84] F. Yin, M. Zhao, Y. Liu, W. Han, and Z. Li, “Effect of Si on growth kinetics of intermetallic compounds during reaction between solid iron and molten aluminum," Trans. Nonferrous Met. Soc. China, vol. 23, no. 2, pp. 556–561, Feb. 2013, doi: 10.1016/S1003-6326(13)62499-1.
- [85] G. Eggeler, W. Auer, and H. Kaesche, “REACTIONS BETWEEN LOW ALLOYED STEEL AND INITIALLY PURE AS WELL AS IRON-SATURATED ALUMINUM MELTS BETWEEN 670 AND 800-DEGREES-C," Z. Met., vol. 77, no. 4, pp. 239–244, 1986.
- [86] Makhlouf M. Makhlouf and Diran Apelian, “Casting Characteristics of Aluminum Die Casting Alloys,” DOE/ID/13716, 792701, Feb. 2002. doi: 10.2172/792701.
- [87] A. Zovi and F. Casarotto, “SILAFONT-36, THE LOW IRON DUCTILE DIE CASTING ALLOY DEVELOPMENT AND APPLICATIONS,” Metall. Ital., 2007, [Online]. Available: https://www.fracturae.com/index.php/aim/article/view/517/488
- [88] Chu, Yeou-Li, Balasubramaniam, Srikanth, Rajan, Rajendra, and Shivpuri, Rajiv, "A Study of the Cast Alloy-Die Surface Interactions in Aluminum Die Casting," vol. T97-075, 1997.
- [89] Yu, Mulong, Chu, Yeou-Li, and Shivpuri, Rajiv, “A Study of Corrosion of Die Materials and Die Coatings in Aluminum Die Casting,” in North American Die Casting Congress, Cleveland, OH, Oct. 1993, vol. T93-072, pp. 191–198.
- [90] M. Movahedi, A. H. Kokabi, and S. M. Seyed Reihani, “Investigation on the bond strength of Al-1100/St-12 roll bonded sheets, optimization and characterization,” Mater. Des., vol. 32, no. 6, pp. 3143–3149, Jun. 2011, doi: 10.1016/j.matdes.2011.02.057.
- [91] M. Roulin and J. W. Luster, “Strength and Structure of Furnace-Brazed Joints between Aluminum and Stainless Steel,” Weld. Res. Suppl., vol. 78, no. 5, pp. 151-155, May 1999.
- [92] J. Vrenken, C. Goos, T. Veldt, and W. Braunschweig, “Fluxless Laser Brazing of Aluminium to Steel,” Join. Automot. Eng., 2009, [Online]. Available: Fluxless Laser Brazing of Aluminium to Steel
- [93] Tosa, Hiroki and Urakami, Akio, “Factors Influencing Die Casting Ejection,” in North American Die Casting Congress, Chicago, IL, Oct. 1972, vol. T72-041.
- [94] "Buhler Evolution Die Casting Machine Brochure.” Buhler. Accessed: May 01, 2021. [Online]. Available: https://www.buhlergroup.com/content/buhlergroup/global/en/products/evolution_die-castingmachineevolution.html
- [95] J. Lu, Y. Song, L. Hua, P. Zhou, and G. Xie, “Effect of temperature on friction and galling behavior of 7075 aluminum alloy sheet based on ball-on-plate sliding test,” Tribol. Int., vol. 140, p. 105872, Dec. 2019, doi: 10.1016/j.triboint.2019.105872.
- [96] C. M. Estey, S. L. Cockcroft, D. M. Maijer, and C. Hermesmann, “Constitutive behaviour of A356 during the quenching operation,” Mater. Sci. Eng. A, vol. 383, no. 2, pp. 245-251, Oct. 2004, doi: 10.1016/j.msea.2004.06.004.
- [97] A. Alankar and M. A. Wells, “Constitutive behavior of as-cast aluminum alloys AA3104, AA5182 and AA6111 at below solidus temperatures,” Mater. Sci. Eng. A, vol. 527, no. 29–30, pp. 7812–7820, Nov. 2010, doi: 10.1016/j.msea.2010.08.056.
- [98] C. H. Cáceres, “A Rationale for the Quality Index of Al-Si-Mg Casting Alloys,” p. 17, 1998.
- [99] F. A. Nichols, “Plastic instabilities and uniaxial tensile ductilities,” Acta Metall., vol. 28, no. 6, pp. 663–673, Jun. 1980, doi: 10.1016/0001-6160(80)90144-3.
- [100] R. Overfelt, S. Bakhtiyarov, and R. Taylor, “Thermophysical properties of A201, A319, and A356 aluminium casting alloys,” High Temp.-High Press., vol. 34, no. 4, pp. 401–409, 2002, doi: 10.1068/htjr052.
- [101] R. Brandt and G. Neuer, “Electrical Resistivity and Thermal Conductivity of Pure Aluminum and Aluminum Alloys up to and above the Melting Temperature," Int. J. Thermophys., vol. 28, no. 5, pp. 1429–1446, Nov. 2007, doi: 10.1007/s10765-006-0144-0.
- [102] J. A. Dantzig and M. Rappaz, Solidification, 1 st. EPFL Press, 2009.
- [103] P. Brancaleon, “Specifications and Standards Development for Enhanced Casting Performance through Examination of Mechanical Properties versus Casting Section Thickness,” in NADCA Transactions, Virtual Event, 2020, p. 7.
9. 저작권:
- 본 자료는 알렉스 먼로의 논문: [논문 제목]을 기반으로 합니다.
- 논문 출처: DOI URL
본 자료는 위 논문을 바탕으로 요약되었으며, 상업적 목적으로 무단 사용하는 것을 금지합니다.
Copyright © 2025 CASTMAN. All rights reserved.