1. 概要:
- タイトル:Simulation of variable thickness aluminum alloy plate casting(可変厚アルミニウム合金板)の鋳造シミュレーション
- 著者:G. S. Reddy, and Md. Hafeez
- 出版年:2024年
- 掲載ジャーナル/学会誌:Journal of Physics: Conference Series
- キーワード:鋳造シミュレーション、熱流、流体流動、冷却曲線、凝固
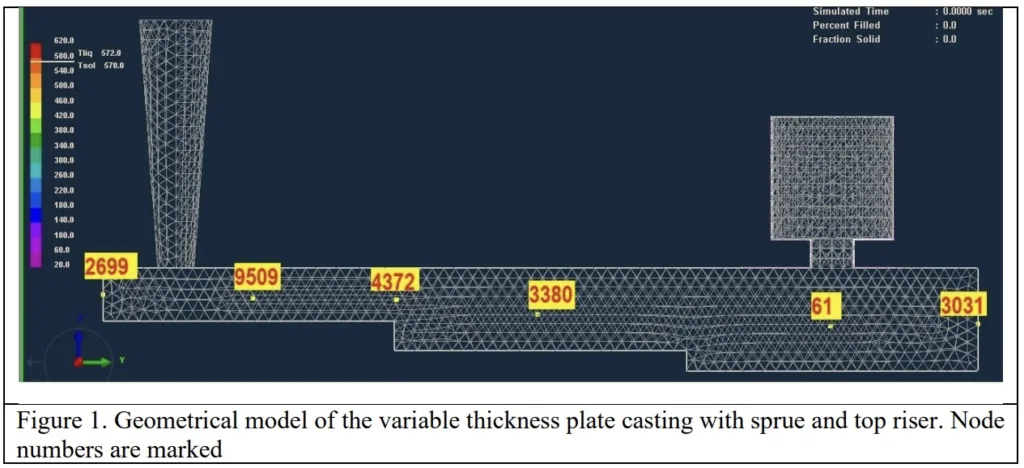
2. 研究背景:
- 研究トピックの社会的/学術的背景:アルミニウム合金は、特に成長著しい電気自動車製造分野において、その多様な特性からますます重要になっています。自動車産業はアルミニウム部品への移行を進めています。
- 既存研究の限界:鋳造シミュレーションの予測と実際の鋳造結果の間には、認識されているギャップが存在します。
- 研究の必要性:本研究は、アルミニウム合金鋳物の凝固プロセスに関する理解を深め、特にマクロ収縮を軽減するために必要です。鋳造プロセスにおける凝固モデルの信頼性と実用性を向上させることを目的としています。
3. 研究目的と研究課題:
- 研究目的:本研究の主な目的は、可変厚アルミニウム合金板の鋳造シミュレーションを調査し、凝固プロセスをより深く理解し、鋳物におけるマクロ収縮を低減する方法を見つけることです。
- 主要な研究課題:本研究は、シミュレーションを可変厚アルミニウム合金板の鋳造プロセスを理解し最適化するために効果的に使用する方法、特に凝固プロセスと収縮軽減に焦点を当てて、暗黙のうちに答えることを目指しています。
- 研究仮説:本研究は、シミュレーションが可変厚アルミニウム合金板鋳物の収縮気孔率を最小限に抑えるまたは排除するために、鋳造プロセスを理解し最適化するための貴重なツールとなり得るという仮説に基づいて行われています。
4. 研究方法
- 研究デザイン:本研究では、有限要素解析を用いたシミュレーションベースのアプローチを採用し、可変厚アルミニウム合金板鋳物の凝固プロセスをモデル化しています。
- データ収集方法:データはシミュレーション結果を通じて収集され、鋳造モデル内の温度分布、冷却曲線、流体流動、および凝固パターンに焦点を当てています。
- 分析方法:分析には、シミュレーション結果の数値的検討が含まれ、凝固プロセスを理解し、潜在的な収縮領域を特定し、鋳造設計の有効性を評価します。研究は、熱伝達、流体流動、および凝固ダイナミクスに焦点を当てています。
- 研究対象と範囲:研究は、Al-13%Si合金と砂型を用いた可変厚板鋳造のシミュレーションに焦点を当てています。幾何学的モデルには、ブラインドトップライザーとオープンスプルーが含まれています。比較分析のために、サイドライザーを備えた代替構成も実装されました。
5. 主な研究結果:
- 主要な研究結果:シミュレーション結果は、可変厚アルミニウム合金板鋳物において収縮気孔率が存在しないことを示しています。最後の凝固イベントは、ライザーとスプルーで観察されます。温度分布と冷却曲線は、望ましい温度勾配に沿った方向凝固を確認しています。
- 統計的/定性的分析結果:シミュレーション結果の定性分析により、以下が明らかになりました。
- 収縮気孔率の欠如:「シミュレーションの結果、収縮気孔率がないことが明らかになりました。」
- 最終凝固位置:「最後の凝固イベントはライザーとスプルーで発生します。」
- 方向凝固:「温度分布と冷却曲線は、望ましい温度勾配に沿った方向凝固を裏付けています。」
- データ解釈:結果は、アルミニウム合金における収縮気孔率を低減する上でのシリコンの役割を強調しています。本研究では、正確なシミュレーション結果を得るためには、温度依存性のある材料特性や初期境界条件など、質の高い入力データが非常に重要であることを強調しています。乱流ダイナミクス、体積対表面積比の弾性率、方向凝固などの要因に焦点を当てたシミュレーションプロセスデータの継続的な監視は、予測モデルを改良するために重要です。
- 図のリスト:
- 図1. スプルーとトップライザーを備えた可変厚板鋳造の幾何学的モデル。
- 図2. 金型キャビティへの液体アルミニウム合金の充填。流体流速ベクトルは、乱流のない一方向の流れを示しています。
- 図3. ライザーキャビティへの充填。観測された最大流体速度は0.621 m/sです。
- 図4. スプルーとライザーに見られる収縮気孔率。
- 図5. 凝固後の温度分布。
- 図6. 冷却曲線は、スプルーからライザーへの方向凝固を示しており、モデルが望ましい温度勾配を促進する有効性を検証しています。
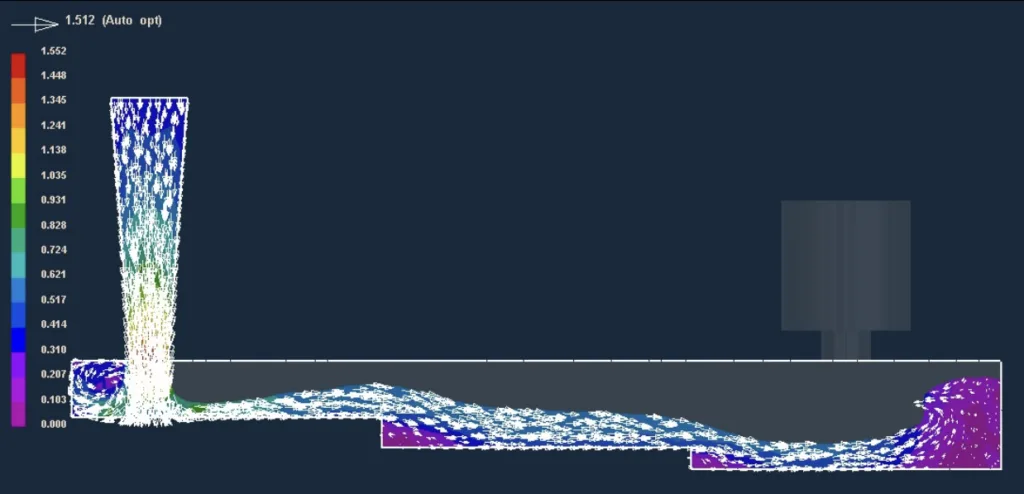
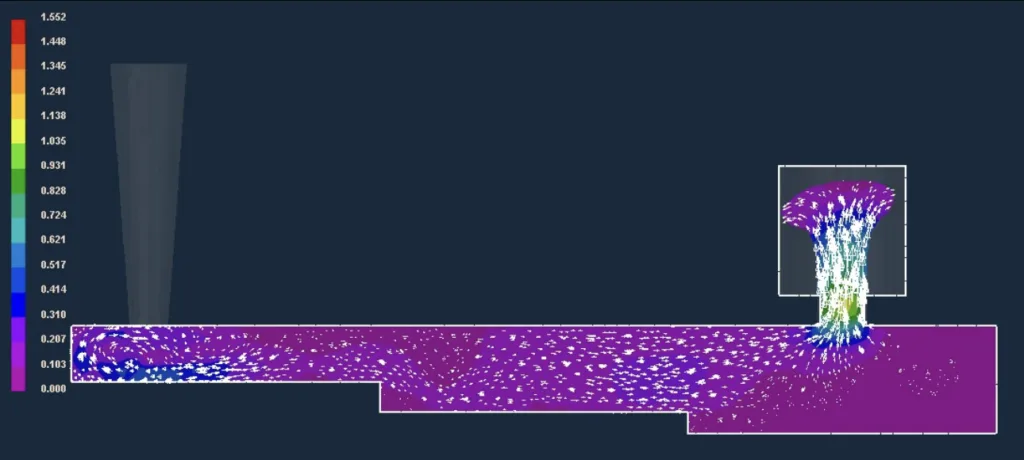
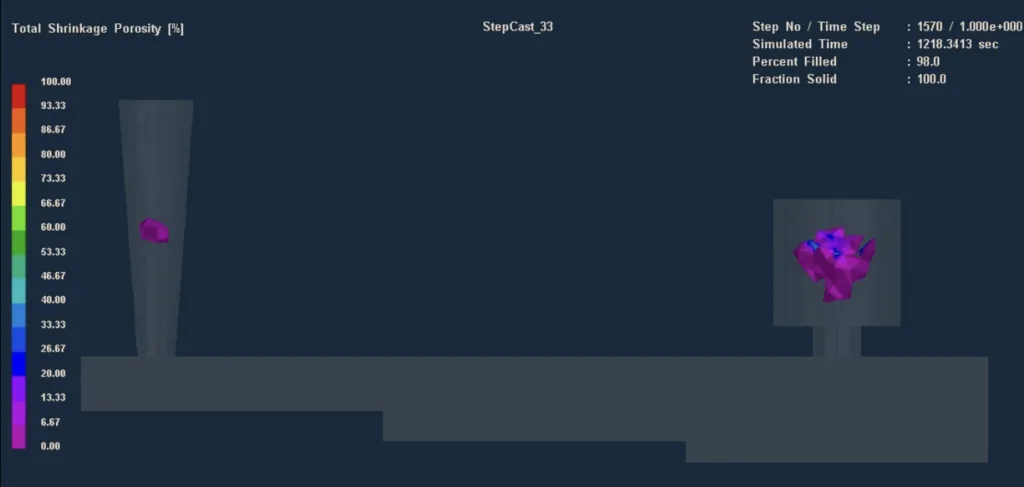
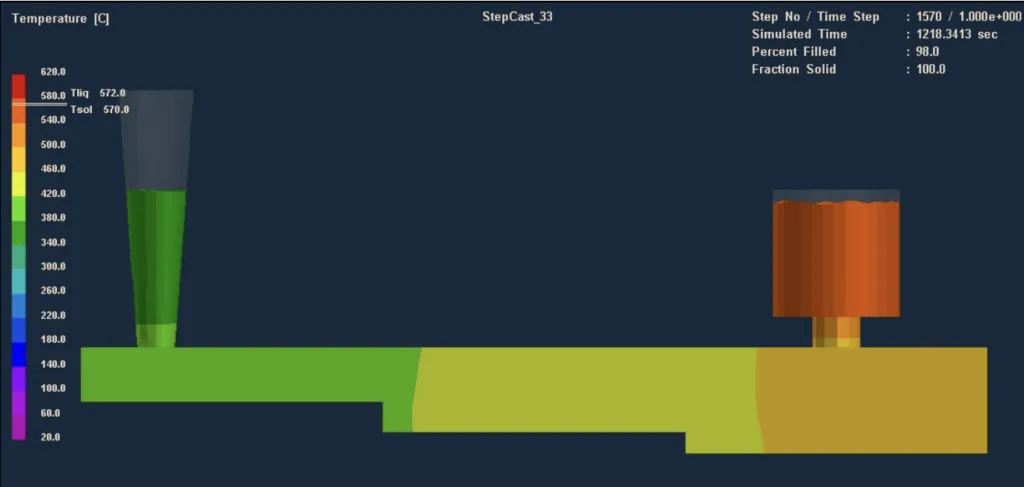
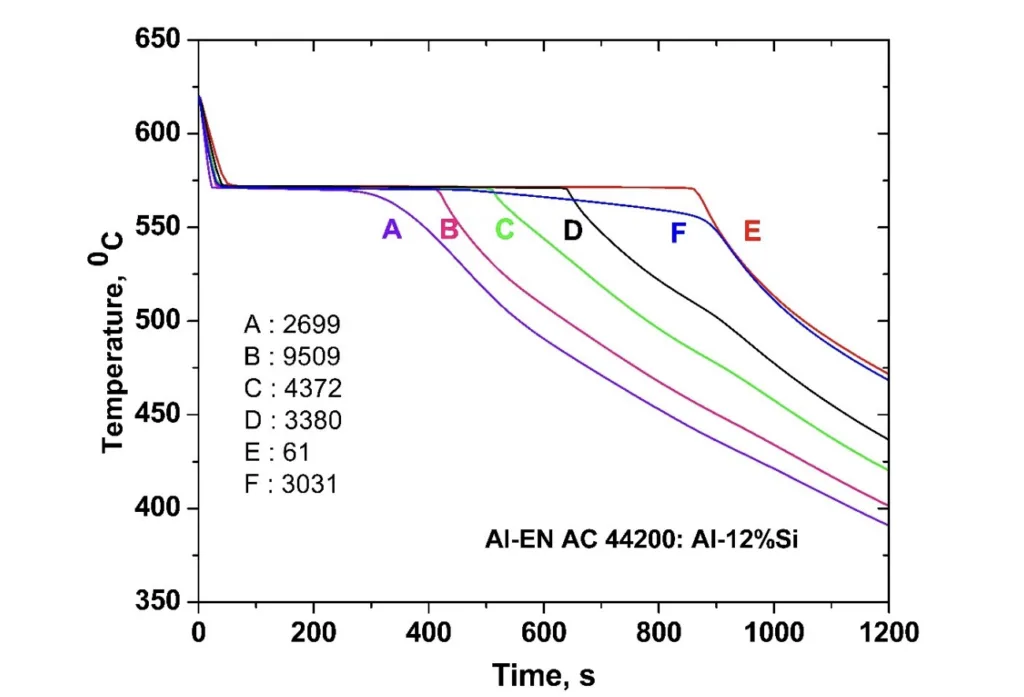
6. 結論と考察:
- 主な結果の要約:可変厚アルミニウム合金板の鋳造シミュレーションは、収縮気孔率がないことを示し、最終凝固はライザーとスプルーで発生しました。シミュレーションは方向凝固も確認しました。
- 研究の学術的意義:本研究は、アルミニウム合金の凝固プロセスに関する理解の進歩に貢献しています。シミュレーションと実際の鋳造結果の間のギャップを埋めることで、凝固モデルの信頼性と適用性を高めます。
- 実用的な意義:本研究は、特にアルミニウム合金鋳物のマクロ収縮を軽減するために、シミュレーションを通じて鋳造プロセスを最適化するための貴重な洞察を提供します。この知見は、アルミニウム部品の採用を増やしている自動車産業にとって特に重要です。
- 研究の限界:本研究は、シミュレーション結果の精度が、温度依存性のある材料特性や初期境界条件などの入力データの品質に大きく依存していることを強調しています。これは、鋳造シミュレーションにおける慎重なデータ管理と検証の必要性を強調しています。
7. 今後のフォローアップ研究:
- フォローアップ研究の方向性:今後の研究では、合金組成、さまざまな鋳造技術、およびシミュレーション手法の進歩の継続的な探求に焦点を当てる必要があります。これにより、さまざまな産業におけるアルミニウム合金の性能がさらに向上し、有用性が拡大します。
- さらなる探求が必要な分野:予測モデルを改良するには、シミュレーションプロセスデータを継続的に監査することが必要です。主な分野には、金型充填中の乱流ダイナミクス、体積対表面積比の弾性率、および方向凝固制御が含まれます。
8. 参考文献:
- G.M. Scamans, N. Birbilis, R.G. Buchheit, “Corrosion of Aluminium and its Alloys", Shreir's Corrosion, Volume 3, 2010, Pages 1974-2010; https://doi.org/10.1016/B978-044452787-5.00095-0
- J. Gilbert Kaufman, and Elwin L. Rooy, “Aluminum alloy castings: Properties, Processes, and applications", 2004 ASM International. pp 1-321.
- Baser, T. A., Umay, E., & Akinci, V. (2022). “New trends in aluminum casting alloys for automotive applications. The Eurasia Proceedings of Science Technology engineering and mathematics, 21, 79-87. https://doi.org/10.55549/epstem.1227541.
- C. Berlanga-Labari, M. V. Biezma-Moraleda, and Pedro J. Rivero, “Corrosion of Cast Aluminum Alloys: A Review", MDPI journal, Metals 2020, 10, 1384; doi:10.3390/met10101384
- Mariem Ben Saada, Mohamed El Mansouri, “Assessment of the effect of 3D printed sand mold thickness on solidification process of AlSi13 casting alloy", International Journal of Advanced Manufacturing Technology, 2021, 114 (5-6), pp.1753-1766. 10.1007/s00170-021-06999-3. HAL-03220855
- Meet Upadhyay, Tharmalingam Sivarupan, Mohamed El Mansori, “3D printing for rapid sand casting- A review", Journal of Manufacturing Processes, 2017, 29, pp.211-220. 10.1016/j.jmapro.2017.07.017. hal-02417595.
- D. Martinez, C. Bate, and G. Manogharan, “Towards functionally graded sand molds for metal casting: Engineering thermo-mechanical properties using 3D sand printing", 2020 The Minerals, Metals & Materials Society, https://doi.org/10.1007/s11837-019-03975-x, Materials properties, ESI Group, 04-June-2013
- Cusato, N.; Nabavizadeh, S.A.; Eshraghi, M. A Review of large-scale simulations of microstructural evolution during alloy solidification. Metals 2023, 13, 1169. https://doi.org/10.3390/met13071169, Academic editors: Changing Fang and Chang Yong Jo.
- Philip King and Guha Manogharan, “Novel experimental method for metal flow analysis using open molds for sand casting”, International Journal of Metal casting/Volume 17, Issue 4, 2023. Pp 2892-2903.
- Bate, C.; King, P.; Sim, J.; Manogharan, G, “A novel approach to visualize liquid aluminum flow to advance casting science, Materials, 2023, 16, 756. https://doi.org/10.3390/ma16020756.
- Griffiths, W. D., & Atkinson, H. V. (1999). The influence of alloy composition on shrinkage porosity formation during the solidification of aluminum alloys. Metallurgical and Materials Transactions A, 30(2), 361-372.
- El-Bealy, M. M., Abd-Elhady, E. A., & Abdel-Rahman, A. M. (2019). Simulation of shrinkage defects in aluminum alloy castings using numerical modeling. Journal of Manufacturing Processes, 41, 72-80.
- GS Reddy, WJ Mascarenhas, JN Reddy, Metallurgical Transactions B 24, 677 -684.
- Cheng Guª, Colin D. Ridgewaya, Emre Cinkilica, Yan Luª, Alan A. Luo a,*
- Olawale Olarewaju Ajibola, et al, "Effects of molding sand permeability and pouring temperatures on properties of cast 6061 aluminum alloy", International Journal of Metals, Volume 2015, Article ID 632021, pp 21-13, http://dx.doi.org/10.1155/2015/632021
- Neuser, M.; Grydin, O.; Andreiev, A.; Schaper, M. Effect of Solidification Rates at Sand Casting on the Mechanical Joinability of a Cast Aluminium Alloy. Metals 2021, 11, 1304. https://doi.org/10.3390/met11081304
- Dash, S.S.; Chen, D. A, “Review on processing-microstructure-property relationships of Al-Si alloys"; Recent advances in deformation behavior, Metals 2023, 13, 609. https://doi.org/10.3390/met13030609.
- For a Livable Climate: Net-Zero Commitments Must Be Backed by Credible Action. Available online: https://www.un.org/en/climatechange/net-zero-coalition (accessed on 20 January 2023).
- Svendsen, A. Aluminum Continues Unprecedented Growth in Automotive Applications. Available online: https://www.lightmetalage.com/news/industry-news/automotive/aluminum-continues-unprecedented-growth-in-automotiveapplications/(accessed on 20 January 2023
- Javidani, M.; Larouche, D. Application of CastAl-Si Alloys in Internal Combustion Engine Components. Int. Mater. Rev. 2014, 59, 132–158. [CrossRef].
- Torres, R.; Esparza, J.; Velasco, E.; Garcia-Luna, S.; Colas, R. Characterisation of an Aluminum Engine Block. Int. J. Microstructure. Mater. Prop. 2006, 1, 129. [CrossRef]
- Czerwinski, F. Thermal Stability of Aluminum Alloys. Materials 2020, 13, 3441. [CrossRef]
- Fan, Y.; Makhlouf, M.M. The Al-Al3Ni Eutectic Reaction: Crystallography and Mechanism of Formation. Metall. Mater. Trans. A 2015, 46, 3808–3812. [CrossRef]
- Czerwinski, F.; Kasprzak, W.; Sediako, D.; Emadi, D.; Shaha, S.; Friedman, J.; Chen, D.L. High-Temperature Aluminum Alloys for Automotive Powertrains; Cast Aluminum AlloysWere Developed with High-Temperature Tensile and Fatigue Strengths to Withstand Elevated-Temperature Applications in Modern Engines. Adv. Mater. Process. 2016, 174, 16–21.
- Geoffrey Sigworth, and Timothy A. Kuhn, “Grain Refinement of Aluminum Casting Alloys", International Journal of Metal casting, September 2007, 115(2):1-12; DOI:10.1007/BF03355416.
- Jun Wang, Shuxian He, and Baode Sun, "Grain refinement of Al-Si alloy (A356) by melt thermal treatment", Journal of Materials Processing Technology, October 2003, 141(1):29-34, DOI:10.1016/S0924-0136(02)01007-5
- Zamani, Mohammadreza, “Al-Si Cast alloys - Microstructure and mechanical properties at ambient and elevated temperatures”, Ph.D. thesis, 2017, Jönköping University, School of Engineering.
- Fracchia, E.; Gobber, F.S.; Rosso, M. "Effect of alloying elements on the Sr modification of Al-Si cast alloys”. Metals 2021, 11, 342. https:// doi.org/10.3390/met11020342.
- Sathyapal Hegde and K. Narayan Prabhu, “Modification of eutectic silicon in Al-Si alloys", J Mater Sci (2008) 43:3009–3027, DOI 10.1007/s10853-008-2505-5, pp 1-19.
- X Cao and J Campbell, "Hard spot defects in aluminum-silicon cast alloys", Shape Casting: The John Campbell Symposium Edited by Murat Tiryakioglu and Paul N. Crepeau TMS (The Minerals, Metals, & Materials Society), 2005 https://www.researchgate.net/publication/287911378
- Anil Saigal, Edwin R. Fuller Jr, “Analysis of stresses in aluminum-silicon alloys", Computational Materials Science, Volume 21, Issue 1, May 2001, Pages 149-158.
- R.B. Gundlach et al, “Thermal fatigue resistance of hypoeutectic aluminum-silicon casting alloys", AFS Trans. (1994)
- Bao Quoc Doan, Dinh Tuyen Nguyen, Minh Nhat Nguyen, Tri Hieu L, Thi Minh Hao Dong, Le Hung Duong, “A Review on Properties and Casting Technologies of Aluminum Alloy in The Machinery Manufacturing", Journal of Mechanical Engineering Research and Developments ISSN: 1024-1752 CODEN: JERDFO Vol. 44, No. 8, pp. 204-217 the Published Year 2021.
- G.S. Reddy and G.R.K. Murthy, "Liquid forging of an aluminum alloy”, Trans of the IIM, 31 (5), 484-487, 1978.
- G.S. Reddy, Ph.D. thesis, “Solidification of metals and alloys under pressure", 1987, Institute of Technology, BHU, Varanasi, India.
- GS Reddy, K Saurabh, DM Krishna, ‘Numerical Simulation Of Directionally Solidified CM247LC High Pressure Turbine Blade', Materials Today: Proceedings 4 (8), 7820-7830.
- GS Reddy, K. Saurabh, R Yedu Krishnan, Numerical Simulation of CM247SX Single Crystal High-Pressure Turbine Vane, Materials Today Proceedings Science Direct, 7837 – 7847.
9. 著作権:
- この資料は、G. S. Reddy, and Md. Hafeez氏の論文:「Simulation of variable thickness aluminum alloy plate casting」に基づいています。
- 論文ソース:doi:10.1088/1742-6596/2837/1/012092
この資料は上記論文に基づいて要約したものであり、商業目的での無断使用は禁止されています。
Copyright © 2025 CASTMAN. All rights reserved.