この論文概要は、Materials, MDPIに掲載された論文「Evaluation of the Microstructure and Properties of As-Cast Magnesium Alloys with 9% Al and 9% Zn Additions」に基づき作成されました。
1. 概要:
タイトル: Evaluation of the Microstructure and Properties of As-Cast Magnesium Alloys with 9% Al and 9% Zn Additions
著者: レホスワフ・トゥズ (Lechosław Tuz)、ヴィート・ノヴァーク (Vít Novák)、フランティシェク・タティチェク (František Tatíček)
発表年: 2025年
掲載ジャーナル: Materials, MDPI
キーワード: マグネシウム合金、機械的特性、微細構造、溶接性、成形、高温、熱伝導率
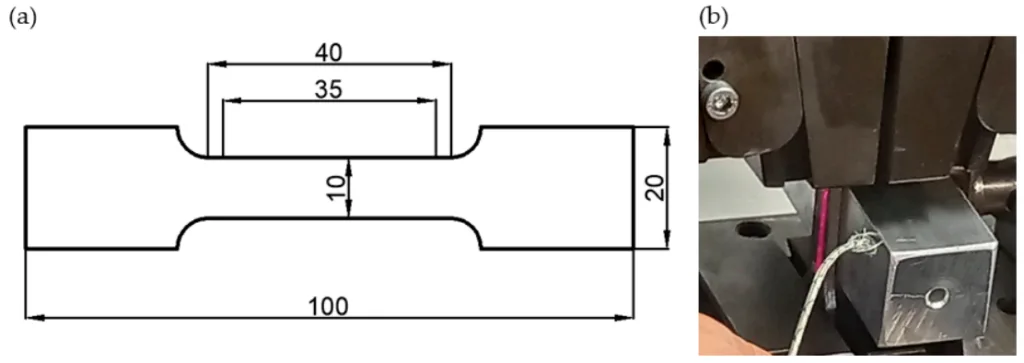
2. 研究背景:
エネルギー消費削減の必要性は、車両の軽量化を要求しており、これは電気自動車の開発とともに、マグネシウム合金を主要な構造材料として再評価させる要因となっています。マグネシウム合金は、優れた機械的特性、自然分解性、そして自動車および航空宇宙産業において、高温環境を含む厚肉部材と薄肉部材の両方への適用可能性が高まっています。しかし、特に砂型鋳造や高圧ダイカストなどの方法で製造された厚肉鋳物において、マグネシウム合金の活用における主な課題は、固有の多孔性です。この多孔性は、機械的特性および塑性特性に悪影響を及ぼし、熱処理の効率を制限します。さらに、これらの合金中に存在する低融点構造成分および相は、さらなる複雑さを引き起こします。したがって、マグネシウム合金の適用を拡大するためには、これらの限界を理解し、緩和することが重要です。
3. 研究目的と研究課題:
本研究は、産業応用に関連する特定の合金添加物を用いて、as-castマグネシウム合金の微細構造と材料特性を評価することを目的としています。特に、アルミニウムと亜鉛を主要な合金元素として使用し、合金特性に及ぼす影響を比較分析します。
主な研究課題:
- 9%アルミニウム(Al)および9%亜鉛(Zn)の添加は、as-castマグネシウム合金の微細構造にそれぞれどのような影響を与えるか?
- これらの合金添加は、常温および高温におけるas-castマグネシウム合金の機械的特性(引張強度、硬度、塑性)にどのような影響を与えるか?
- これらのマグネシウム合金の熱伝導率は、温度および合金組成によってどのように変化するか?
研究仮説:
本研究では、マグネシウム合金にアルミニウムまたは亜鉛を高濃度で添加すると、低融点相の形成が促進され、加工中の高温割れ感受性が増加すると仮定します。また、研究は、使用条件および溶接などの熱処理プロセス下での挙動を理解するために、これらの合金の温度依存性特性の変化を調査します。
4. 研究方法論
研究デザイン: 本研究では、砂型鋳型で鋳造された2種類のマグネシウム合金、すなわちMg-Al-Zn(約9% Al、0.5% Zn、残部Mg)およびMg-Zn-Al(約9% Zn、1% Al、残部Mg)合金を比較分析する実験的デザインを採用しました。両合金とも、後続の熱処理なしにas-cast状態で試験されました。
データ収集方法: 包括的な特性評価技術セットが活用されました。
- 顕微鏡観察: 光学顕微鏡(LM)および走査型電子顕微鏡(SEM)を使用して、エッチングされた試料の微細構造を観察しました。エネルギー分散型分光法(SEM-EDS)を使用して、相の識別および元素マッピングを実行しました。
- 熱伝導率測定: 熱伝導率係数は、ASTM E1461規格に準拠して、25℃から200℃の範囲の温度で測定されました。
- 引張試験: 静的引張試験は、常温および高温(120℃、150℃、180℃、240℃)で実施されました。試料は、誘導加熱素子を用いた接触加熱方式で加熱されました。伸びは、レーザー伸び計を使用して測定されました。
- 硬さ試験: ビッカース硬さ測定(HV10)を実施して、両合金の硬さを評価しました。
- 破面解析: 引張試験後の試料の破断面をSEMを用いて観察し、破壊メカニズムを分析しました。
分析方法: 収集されたデータは、定性的および定量的な方法を用いて分析されました。微細構造的特徴および相組成は、顕微鏡観察およびEDSを通じて識別されました。統計分析は、機械的および熱的特性データに適用されました。破面解析は、合金の破壊挙動に関する洞察を提供しました。
研究対象と範囲: 本研究は、砂型鋳型で重力鋳造により準備された2つの特定のマグネシウム合金組成、Mg-Al-ZnおよびMg-Zn-Alに焦点を当てました。範囲は、as-cast状態と最大240℃までの微細構造、熱伝導率、および引張特性評価に限定されました。
5. 主な研究結果:
主な研究結果:
- 微細構造: 両合金とも、鋳造材料の典型的な樹枝状晶微細構造を示しました。樹枝状晶間領域には、低融点共晶相、金属間化合物、およびラーベス相が存在しました。Mg-Al-Zn合金は、樹枝状晶間領域に局所的な多孔性を示しました(図2および3)。Mg-Al-Zn合金では、γ-Mg17Al12相が粒界で主に観察され、Mg-Zn-Al合金では、γ-Mg17Al12相およびβ-MgZn2相の両方が存在する可能性が高いことが示されました(図4および5)。
- 熱伝導率: 熱伝導率係数は、両合金とも最大200℃までの温度上昇に対して比較的一定の値を維持しました。Mg-Zn-Al合金は、Mg-Al-Zn合金(約49 W/mK)と比較して、著しく高い熱伝導率(約77 W/mK)を示しました(図8、表4)。
- 引張特性: 引張強度(Rm)は、初期には両合金とも温度上昇とともに増加し、高温で最大値に達しました。Mg-Zn-Al合金の場合、最大引張強度121 MPaは150℃で観察され、Mg-Al-Zn合金は180℃で最大122 MPaに達しました(図9、表5)。伸びは、as-cast状態では両合金とも低いままでした。
- 硬さ: Mg-Al-Zn合金は、Mg-Zn-Al合金(46 HV10)と比較して、著しく高いビッカース硬さ(83 HV10)を示しました(表6)。
統計的/定性的分析結果: EDS分析により、樹枝状晶間領域に合金元素が存在することが確認され、主要な相が特定されました。引張試験データは、強度および伸びにおいて温度依存性のある傾向を示しました。熱伝導率測定は、熱伝達特性に関する定量的な値を提供しました。破面解析は、両合金とも脆性粒界破壊を示唆しました(図10および11)。
データ解釈: 結果は、合金元素、特にAlとZnが凝固中に樹枝状晶間領域に偏析し、低融点相を形成することを示唆しています。亜鉛添加は、ラーベス相の形成に起因して、熱伝導率を大幅に向上させます。高温は、初期には原子の移動度増加により引張強度を向上させますが、塑性を低下させる可能性があります。Mg-Al-Zn合金の多孔性とMg-Zn-Al合金の脆性亜鉛リッチ相の形成は、それらの機械的挙動および潜在的な溶接性に影響を与えます。
図リスト:
- 図1. 引張試験用試料(a)と加熱装置(b)、LabTest Model 5.100SP1、試験に使用。
- 図2. 合金の断面マクロ構造:(a)Mg-Al-Zn、(b)Mg-Zn-Al。
- 図3. Mg-Al-Zn合金の多孔性付近の微細構造。
- 図4. Mg-Al-Zn合金の微細構造。(a-d)光学顕微鏡、(e,f)SEM像。
- 図5. Mg-Zn-Al合金の微細構造。(a-d)光学顕微鏡、(e,f)SEM像。
- 図6. Mg-Al-Zn合金の元素分布マップ。
- 図7. Mg-Zn-Al合金の元素分布マップ。
- 図8. 温度関数における熱伝導率係数の変化。
- 図9. (a)Mg-Al-Znおよび(b)Mg-Zn-Al合金の機械的特性の変化。
- 図10. 試料92(a,b)および96(c,d)の破面形態。
- 図11. 試料L2(a,b)およびL6(c,d)の破面形態。
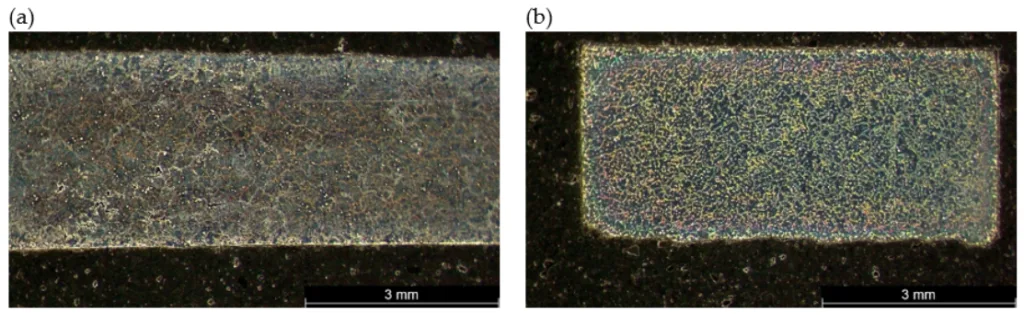
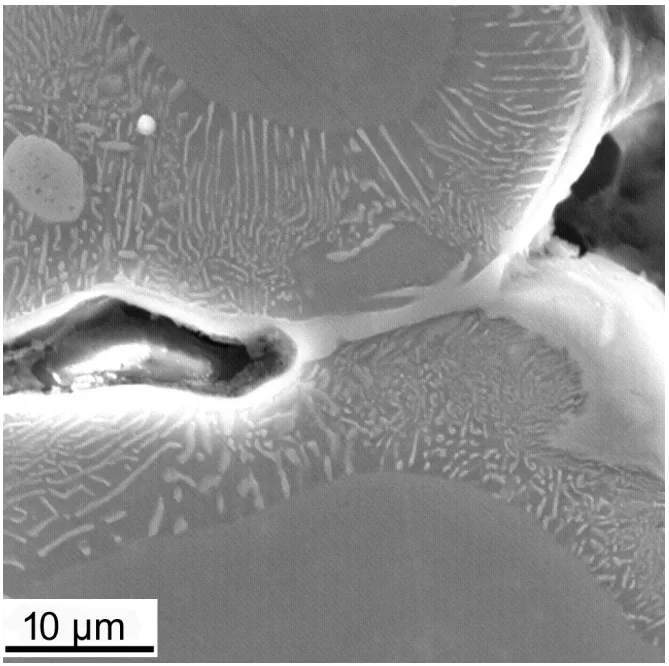
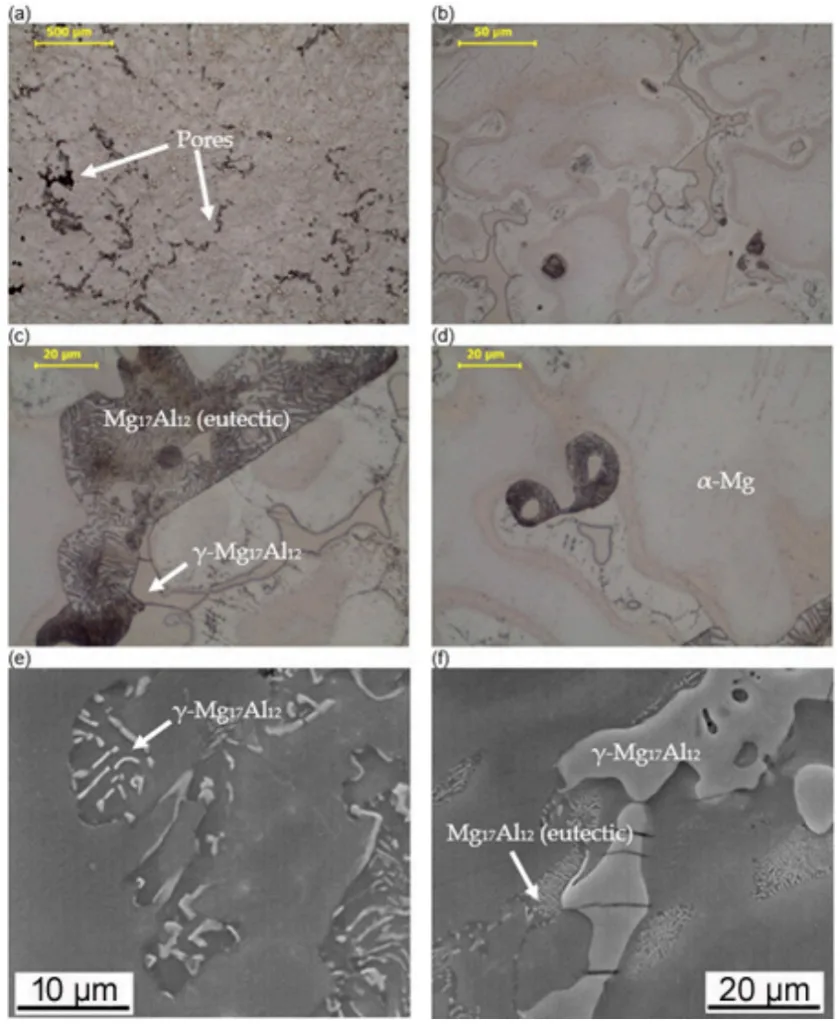
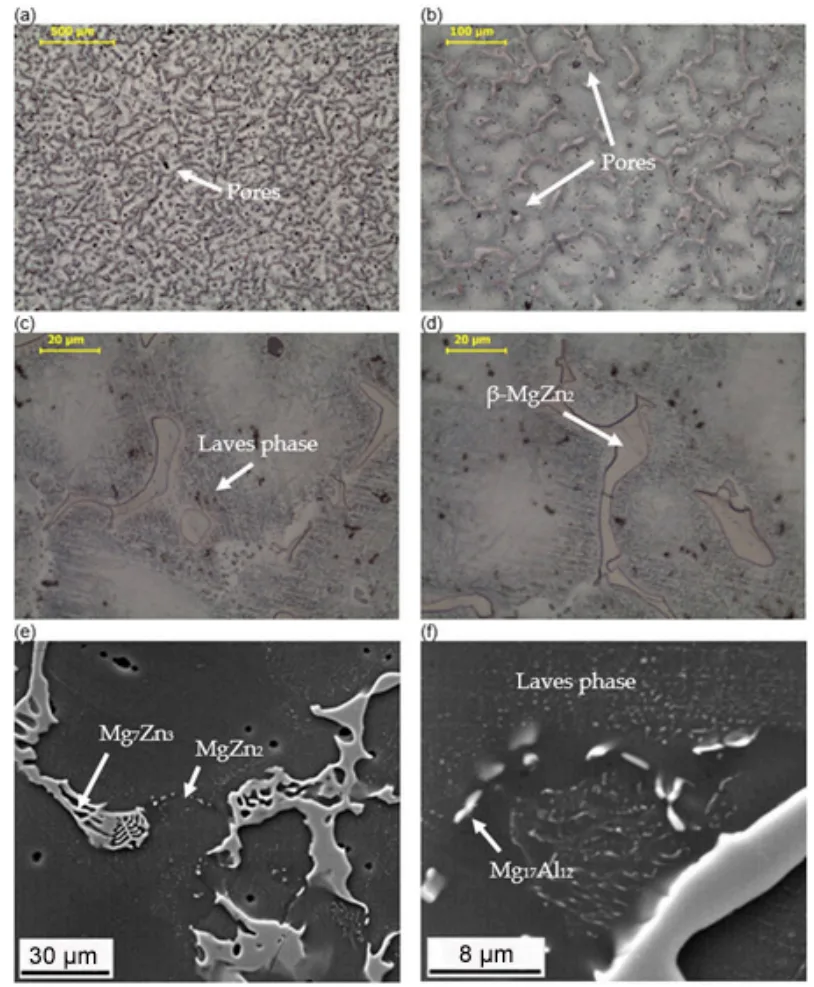
6. 結論と考察:
主な結果の要約: 本研究は、as-cast Mg-Al-ZnおよびMg-Zn-Al合金の両方が、合金元素が樹枝状晶間領域に集中した樹枝状晶微細構造を示すことを示しています。9%の亜鉛を含むMg-Zn-Al合金は、Mg-Al-Zn合金(9%アルミニウム)と比較して優れた熱伝導率を示しますが、硬度は低くなります。両合金とも高温で最大引張強度を示しますが、低融点相の存在により高温割れを起こしやすいです。
研究の学術的意義: 本研究は、as-castマグネシウム合金の微細構造と特性に対するアルミニウムおよび亜鉛合金添加の比較効果に関する貴重な洞察を提供します。これは、産業的に関連性の高いこれらの合金システムにおける相形成、熱的挙動、および機械的応答のより深い理解に貢献します。
実用的な意味: 研究結果は、マグネシウム合金のダイカストおよび溶接における材料選択と加工に実用的な意味を持ちます。Mg-Zn-Al合金の高い熱伝導率は、効率的な放熱が必要な用途で有利となる可能性があります。しかし、両合金とも高温割れに対する感受性があることは、特に自動車および航空宇宙産業向けの大型鋳物において、部品の健全性を確保するために、予熱を含む鋳造および溶接パラメータを慎重に制御する必要があることを強調しています。溶接中の割れを軽減するために、最大引張強度範囲(150〜180℃)での予熱が推奨されます。
研究の限界: 本研究は、as-cast状態の2つの特定の合金組成に限定されています。溶接性試験は実施されておらず、これらの合金に対する熱処理効果および溶接パラメータの最適化を調査するための追加研究が必要です。
7. 今後のフォローアップ研究:
今後の研究方向: 今後の研究は、以下の点に焦点を当てる必要があります。
- 熱処理および人工時効条件下でのマグネシウムマトリックス内の析出挙動および相変態の詳細な分析。
- 最適な溶接パラメータおよび高温割れ防止戦略を決定するための両合金の包括的な溶接性試験。
さらなる探求が必要な領域:
- 高温割れ感受性を最小限に抑えるための鋳造および溶接中の予熱温度および冷却速度の最適化の研究。
- これらのマグネシウム合金の微細構造、特性、および溶接性に対する微量合金元素および不純物レベルの影響に関するさらなる探求。
8. 参考文献:
- [1] Zeng, X.; Yi, S. Deformation Mechanisms of Magnesium Alloys with Rare-Earth and Zinc Additions under Plane Strain Compression. Materials 2024, 17, 33. CrossRef
- [2] Cheng, M.; Jia, X.; Zhang, Z. Prediction of Mechanical Properties of Rare-Earth Magnesium Alloys Based on Convolutional Neural Networks. Materials 2024, 17, 4956. CrossRef PubMed
- [3] Sharma, S.K.; Gajević, S.; Sharma, L.K.; Pradhan, R.; Miladinović, S.; Ašonja, A.; Stojanović, B. Magnesium-Titanium Alloys: A Promising Solution for Biodegradable Biomedical Implants. Materials 2024, 17, 5157. CrossRef PubMed
- [4] Zhao, D.; Lü, S.; Guo, W.; Li, S.; Li, J.; Guo, G.; Guo, W.; Wu, S. Effects of Mg17Al12 phase on microstructure evolution and ductility in the AZ91 magnesium alloy during the continuous rheo-squeeze casting-extrusion process. J. Mater. Sci. Technol. 2024, 191, 63–79. CrossRef
- [5] Mashoufi, K.; Garmroodi, P.; Mirzakhani, A.; Assempour, A. Cyclic contraction-expansion extrusion (CCEE): An innovate severe plastic deformation method for tailoring the microstructure and mechanical properties of magnesium AZ91 alloy. J. Mater. Res. Technol. 2023, 26, 8541–8554. CrossRef
- [6] Chai, F.; Ma, Z.; Han, X.; Hu, X.; Chang, Z.; Zhou, J. Effect of strain rates on mechanical behavior, microstructure evolution and failure mechanism of extruded-annealed AZ91 magnesium alloy under room-temperature tension. J. Mater. Res. Technol. 2023, 27, 4644–4656. CrossRef
- [7] Wang, R.; Yan, F.; Sun, J.; Xing, W.; Li, S. Microstructural Evolution and Mechanical Properties of Extruded AZ80 Magnesium Alloy during Room Temperature Multidirectional Forging Based on Twin Deformation Mode. Materials 2024, 17, 5055. CrossRef
- [8] Venkatesh, R.; Hossain, I.; Mohanavel, V.; Soudagar, M.E.M.; Alharbi, S.A.; Al Obaid, S. Analysis and optimization of machining parameters of AZ91 alloy nanocomposite with the Influences of nano ZrO2 through vacuum diecast process. Heliyon 2024, 10, e34931. CrossRef
- [9] Sadiq, T.O.; Sudin, I.; Alsakkaf, A.; Idris, J.; Fadil, N.A. Effect of homogenization heat treatment on the microstructure of AZ91 magnesium alloy at different temperatures and ageing times. J. Eng. Res. 2023, 11, 212–218. CrossRef
- [10] Ganguly, S.; Chaubey, A.; Sahoo, R.; Kushwaha, A.; Basu, A.; Majhi, J.; Gupta, M. Influence of ultrasonic shot peening on the microstructure and impression creep performance of squeeze-cast AZ91 alloy reinforced with graphene nanoplatelets. J. Alloys Compd. 2023, 938, 168640. CrossRef
- [11] Kandemir, S.; Bohlen, J.; Dieringa, H. Influence of recycled carbon fiber addition on the microstructure and creep response of extruded AZ91 magnesium alloy. J. Magnes. Alloys 2023, 11, 2518–2529. CrossRef
- [12] Motavallian, P.; Rabiee, S.M.; Aval, H.J. Effect of solution treatment of AZ91 alloy on microstructure, mechanical properties and corrosion behavior of friction stir back extruded AZ91/bioactive glass composite. J. Mater. Res. Technol. 2023, 25, 6992–7007. CrossRef
- [13] Cui, P.-X.; Hu, M.-L.; Ji, Z.-S.; Wang, Y.; Guo, Y.; Xu, H.-Y. Effect of La/Nd ratio on microstructure and tensile properties of AZ91-RE alloys. Trans. Nonferrous Met. Soc. China 2024, 34, 1456–1469. CrossRef
- [14] Luo, H.; Li, J.; Wang, Y.; Ye, J.; Zhang, Z.; Guan, B.; Chen, X.; Zheng, K.; Pan, F. Influence of Ti particle sizes on microstructure and mechanical behaviors of AZ91-Ti composites under different loading paths. Mater. Sci. Eng. A 2023, 886, 145723. CrossRef
- [15] Mehdipour, M.; Aval, H.J. Effect of friction stir back extrusion rotational speed on microstructure, mechanical properties, and corrosion behaviour of AZ91-Ca alloy. J. Mater. Res. Technol. 2023, 25, 4441–4456. CrossRef
- [16] Motavallian, P.; Rabiee, S.M.; Aval, H.J. Investigation of microstructure and corrosion behavior of AZ91/64SiO2-31CaO-5P2O5 composite wire fabricated by friction stir back extrusion. Surf. Coat. Technol. 2023, 464, 129451. CrossRef
- [17] Patil, H.; Marodkar, A.; Ghosh, A.; Borkar, H. Effect of Ca addition on the microstructure and creep behaviour of AZ91 Mg alloy. Mater. Today Proc. 2023, in press. CrossRef
- [18] Jin, G.; Xiao, M.; Liu, F.; Liu, W.; Chen, Y. Microstructure and mechanical properties of hybrid hexagonal BN nanotube and nanoplatelet reinforced AZ91 Mg matrix composites. J. Mater. Res. Technol. 2024, 32, 1509–1520. CrossRef
- [19] Xiao, P.; Gao, Y.; Sheng, Y.; Yang, C.; Liu, Z.; Yi, Y.; Wu, W.; Zhao, Q.; Gupta, M. Improving wear and corrosion resistances of Mg2Si/AZ91 composites via tailoring microstructure and intrinsic properties of Mg2Si induced by Sb modification. J. Magnes. Alloys 2023, in press. CrossRef
- [20] Milan, S.; Branislav, H.; Martina, J.; Jana, P. Microstructure evolution and corrosion mechanism of AZ91 magnesium alloy in chloride environments for commercial purposes prepared by Ohno continuous casting. Transp. Res. Procedia 2023, 74, 530–537. CrossRef
- [21] Shabestari, S.G.; Ghaniabadi, E. Effect of Ca and rotation speed on microstructure and solidification parameters of AZ91 magnesium alloy produced by semi-solid casting through rotating container process. Trans. Nonferrous Met. Soc. China 2023, 33, 396–408. CrossRef
- [22] Yang, H.; Cui, J.; Zhou, Y.; Tan, J.; Chen, X.; Huang, G.; Zheng, K.; Jin, Y.; Jiang, B.; Pan, F. Microstructures and the improved mechanical properties of AZ91 alloy by incorporating Ti-6Al-4V particles. J. Mater. Res. Technol. 2023, 26, 7340–7353. CrossRef
- [23] Fan, W.; Bai, Y.; Li, T.; Hao, H.; Zhang, X. Effect of hard-plate rolling and annealing treatment on the microstructure and mechanical properties of NbB2 particle-reinforced AZ91 composite. J. Magnes. Alloys 2024, in press. CrossRef
- [24] Zheng, Z.-L.; Deng, K.-K.; Nie, K.-B.; Wang, C.-J.; Xu, C.; Shi, Q.-X. Microstructure and mechanical properties of freeze-casted in-situ ABOW@Al2O3/AZ91 composites. Ceram. Int. 2024, 50, 42015–42025. CrossRef
- [25] Du, Z.-Q.; Deng, K.-K.; Nie, K.-B.; Wang, C.-J.; Xu, C.; Shi, Q.-X. Preparation, microstructure, and mechanical properties of SiCp/AZ91 magnesium matrix laminar material. Mater. Sci. Eng. A 2024, 891, 145977. CrossRef
- [26] Trojanová, Z.; Gärtnerová, V.; Jäger, A.; Námešný, A.; Chalupová, M.; Palček, P.; Lukáč, P. Mechanical and fracture properties of an AZ91 Magnesium alloy reinforced by Si and SiC particles. Compos. Sci. Technol. 2009, 69, 2256–2264. CrossRef
- [27] Yin, C.; Shen, J.; Hu, S.; Zhang, Z. Microstructure and mechanical properties of AZ91 magnesium alloy fabricated by multi-layer and multi-pass CMT based WAAM technique. Results Eng. 2023, 18, 101065. CrossRef
- [28] Srinivasan, S.; Bharath, R.R.; Atrens, A.; Srinivasan, P.B. Microstructure and mechanical properties of welds of AZ31B magnesium alloy produced by different gas tungsten arc welding variants. Def. Technol. 2024, in press. CrossRef
- [29] Braszczyńska-Malik, K.; Mróz, M. Gas-tungsten arc welding of AZ91 magnesium alloy. J. Alloys Compd. 2011, 509, 9951–9958. CrossRef
- [30] Sunil, B.R.; Reddy, G.P.K.; Mounika, A.; Sree, P.N.; Pinneswari, P.R.; Ambica, I.; Babu, R.A.; Amarnadh, P. Joining of AZ31 and AZ91 Mg alloys by friction stir welding. J. Magnes. Alloys 2015, 3, 330–334. CrossRef
- [31] Kumar, S.; Vigneshwar, M.; Selvamani, S.; Prakash, A.; Hariprasath, P. The Comparative Analysis on Friction Stir Welded and Gas Tungsten Arc Welded AZ91 Grade Magnesium Alloy Butt Joints. Mater. Today Proc. 2017, 4, 6688–6696. CrossRef
- [32] Tuz, L.; Kołodziejczak, P.; Kolasa, A. Structure of butt joint of as-cast magnesium alloys. Weld. Int. 2015, 30, 43-47. CrossRef
- [33] Asgari, A.; Delavar, H.; Sedighi, M. Microstructure and surface integrity of machined AZ91 magnesium alloy. J. Mater. Res. Technol. 2023, 22, 735–746. CrossRef
- [34] Swetha Chowdary, V.; Ravikumar, D.; Anand Kumar, S.; Kondaiah, V.V.; Ratna Sunil, B. Influence of heat treatment on the machinability and corrosion behavior of AZ91 Mg alloy. J. Magnes. Alloys 2018, 6, 52–58. CrossRef
- [35] Marakini, V.; Srinivasa Pai, P.; D’Mello, G. Effect of liquid nitrogen assisted milling on AZ91 magnesium alloy. Results Eng. 2024, 22, 102207. CrossRef
- [36] Marodkar, A.S.; Patil, H.; Chavhan, J.; Borkar, H. Effect of gravity die casting, squeeze casting and extrusion on microstructure, mechanical properties and corrosion behaviour of AZ91 magnesium alloy. Mater. Today Proc. 2023, in press. CrossRef
- [37] Lü, Y.; Wang, Q.; Ding, W.; Zeng, X.; Zhu, Y. Fracture behavior of AZ91 magnesium alloy. Mater. Lett. 2000, 44, 265–268. CrossRef
- [38] Sovík, J.; Kajánek, D.; Pastorek, F.; Štrbák, M.; Florková, Z.; Jambor, M.; Hadzima, B. The Effect of Mechanical Pretreatment on the Electrochemical Characteristics of PEO Coatings Prepared on Magnesium Alloy AZ80. Materials 2023, 16, 5650. CrossRef PubMed
- [39] ASTM E1461-13; Standard Test Method for Thermal Diffusivity by the Flash Method. ASTM International: West Conshohocken, PA, USA, 2022.
- [40] Alrasheedi, N.H.; Ataya, S.; Seleman, M.M.E.-S.; Ahmed, M.M.Z. Tensile Deformation and Fracture of Unreinforced AZ91 and Reinforced AZ91-C at Temperatures up to 300 °C. Materials 2023, 16, 4785. CrossRef PubMed
- [41] Lin, Z.; Sheng, D.; Fang, Y.; Xiong, K.; Song, Y. Experimental and Numerical Investigation of the Tensile and Failure Response of Multiple-Hole-Fiber-Reinforced Magnesium Alloy Laminates under Various Temperature Environments. Materials 2023, 16, 5573. CrossRef
9. 著作権:
*この資料は、Lechosław Tuz、Vít Novák、およびFrantišek Tatíčekの論文「Evaluation of the Microstructure and Properties of As-Cast Magnesium Alloys with 9% Al and 9% Zn Additions」に基づいています。
*論文ソース:https://doi.org/10.3390/ma18010010
この資料は上記の論文に基づいて要約されており、商業目的での無断使用は禁止されています。
Copyright © 2025 CASTMAN. All rights reserved.