1.概要:
- 論文タイトル: 電気自動車とハイブリッド電気自動車のための空冷式バッテリー熱管理システムに関するレビュー
- 著者: Gang Zhao, Xiaolin Wang, Michael Negnevitsky, Hengyun Zhang
- 掲載年: 2021年
- 掲載誌: Journal of Power Sources
- キーワード: 電気自動車、リチウムイオン電池、空冷、バッテリー熱管理システム、レビュー
2. 研究背景:
本論文は、1900年代以降の温室効果ガス(GHG)濃度の上昇、特に内燃機関(ICE)による化石燃料の燃焼が主な原因であることを指摘しています。輸送部門はこれらの排出量に大きく寄与しており、温室効果ガス排出量削減と地球温暖化対策として、電気自動車(EV)とハイブリッド電気自動車(HEV)が注目されています。EVとHEVのパフォーマンスと寿命においてバッテリーの熱管理が重要な役割を果たすことが強調されています。
リチウムイオン電池は、高い比エネルギー密度と出力密度、軽量設計、長いサイクル寿命、比較的低い自己放電率などの利点から、EVとHEVで広く使用されています。しかし、リチウムイオン電池は熱暴走を起こしやすく、特定の条件下では火災や爆発の危険性があるという欠点も指摘されています。そのため、効果的なバッテリー熱管理システム(BTMS)が必要不可欠です。既存の研究では、液冷、相変化材料(PCM)、ヒートパイプなどが一般的なBTMSアプローチとして挙げられていますが、EVとHEVにおける空冷式BTMSに関する包括的なレビューは不足しているとして、本研究が実施されました。
3. 研究目的と研究課題:
主な目的は、EVとHEVにおける空冷式BTMSを包括的にレビューすることです。重要な研究課題としては、以下のような点が考えられます。
- リチウムイオン電池をEVとHEVに使用する利点と欠点は何ですか?
- リチウムイオン電池における発熱メカニズムは何ですか?過剰な発熱の結果(熱劣化、熱暴走)は何ですか?
- 空冷式BTMSの基本的な設計原則は何ですか?
- 空冷式BTMSのパフォーマンスを向上させるために、どのような革新的な設計改良が実施されてきましたか?(バッテリーパックレイアウトの最適化、冷却チャネル設計の革新、改良された吸気/排気構成、高度な熱伝導材料の使用など)
- 空冷式BTMSのパフォーマンスと安全性を向上させるための将来の研究方向と潜在的な解決策は何ですか?
4. 研究方法:
本研究は、文献レビューの方法論を用いています。著者らは、空冷式BTMS、リチウムイオン電池、EV、HEVに関連するキーワードを用いて、Scopus、Web of Science、IEEE Xploreなどの関連データベースを体系的に検索したと思われます。収集された文献は、傾向の特定、さまざまな設計アプローチの評価、既存の空冷式BTMS技術のパフォーマンスと限界の評価を行うために分析されました。レビューには、実験的研究と計算流体力学(CFD)シミュレーションから得られた定量的データ(温度分布、発熱率など)が含まれている可能性があります。
5. 主要な研究結果(部分的なテキストに基づく):
部分的なテキストから、研究の主要な結果の一部がわかります。
- 発熱メカニズム: 論文では、リチウムイオン電池における発熱メカニズムについて論じており、可逆的および不可逆的な発熱について説明していると考えられます。これらのメカニズムは、セル電流、充電状態(SOC)、温度、内部抵抗などの要因と関連付けられています。発熱と温度上昇を支配する方程式が提示され、議論されている可能性があります。
- 熱暴走: 熱暴走の深刻な結果(火災や爆発を含む)が強調されています。熱暴走につながる根本的な化学的および物理的プロセスが説明されていると考えられます。
- 空冷式BTMS設計: 空冷式BTMSの基本原理が記述されており、受動冷却システムと能動冷却システムが区別されていると考えられます。受動システムは自然空気の流れに依存する一方、能動システムは冷却を強化するためにファンやブロワーを使用します。
- 設計改良: 部分的なテキストは、空冷式BTMSのパフォーマンスを向上させることを目的としたいくつかの革新的な設計改良についてレビューしていることを示唆しています。これらの改良は、おそらく次のようなカテゴリに分類されます。
- バッテリーパック設計: 気流と放熱を改善するために、パック内のバッテリーセルの配置と間隔を最適化すること。さまざまな配置(並列、直列、スタガードなど)が比較されます。
- 冷却チャネル設計: 熱伝達効率を向上させるために、冷却チャネルの形状と寸法を変更すること。さまざまなチャネル形状(Z型、U型など)について議論されています。
- 吸気/排気設計: 気流分布を改善し、圧力降下を低減するために、空気の吸気口と排気口の位置と構成を最適化すること。
- 熱伝導材料: バッテリーセルから冷却空気への効率的な熱伝達を促進するために、熱伝導率の高い材料を使用すること。
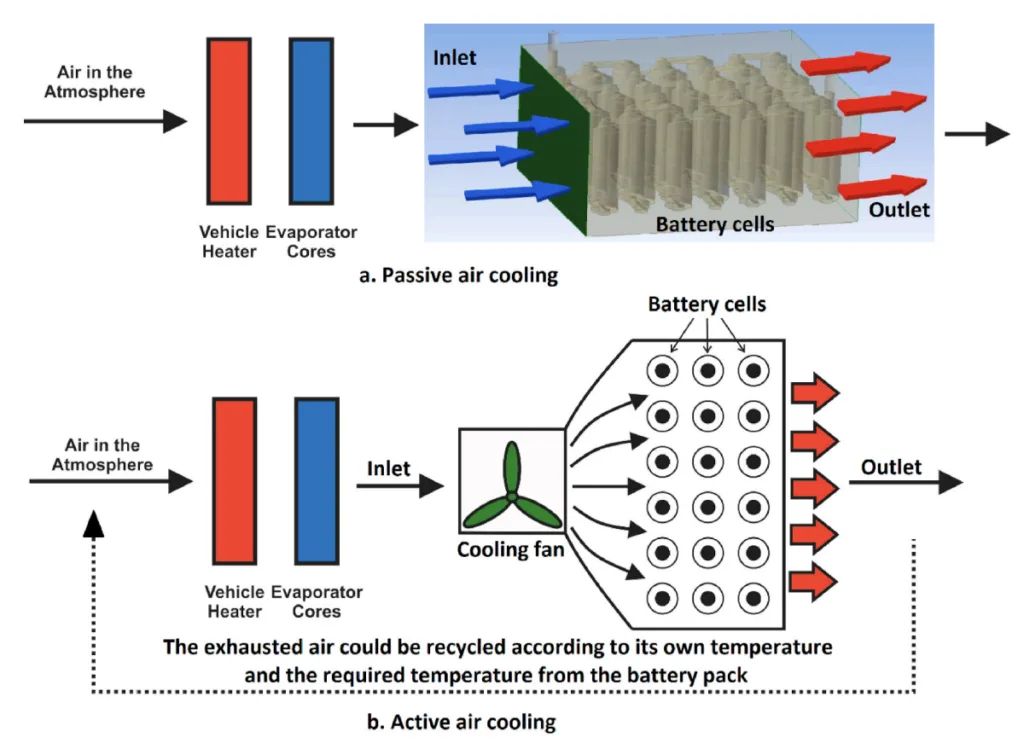
![Fig. 2. Three-stack battery pack with stagger-arranged Lithium-ion battery cells on each stack (Ref. [158]).](https://castman.co.kr/wp-content/uploads/Fig.-2.-Three-stack-battery-pack-with-stagger-arranged-Lithium-ion-battery-cells-on-each-stack-Ref.-158-1024x449.webp)
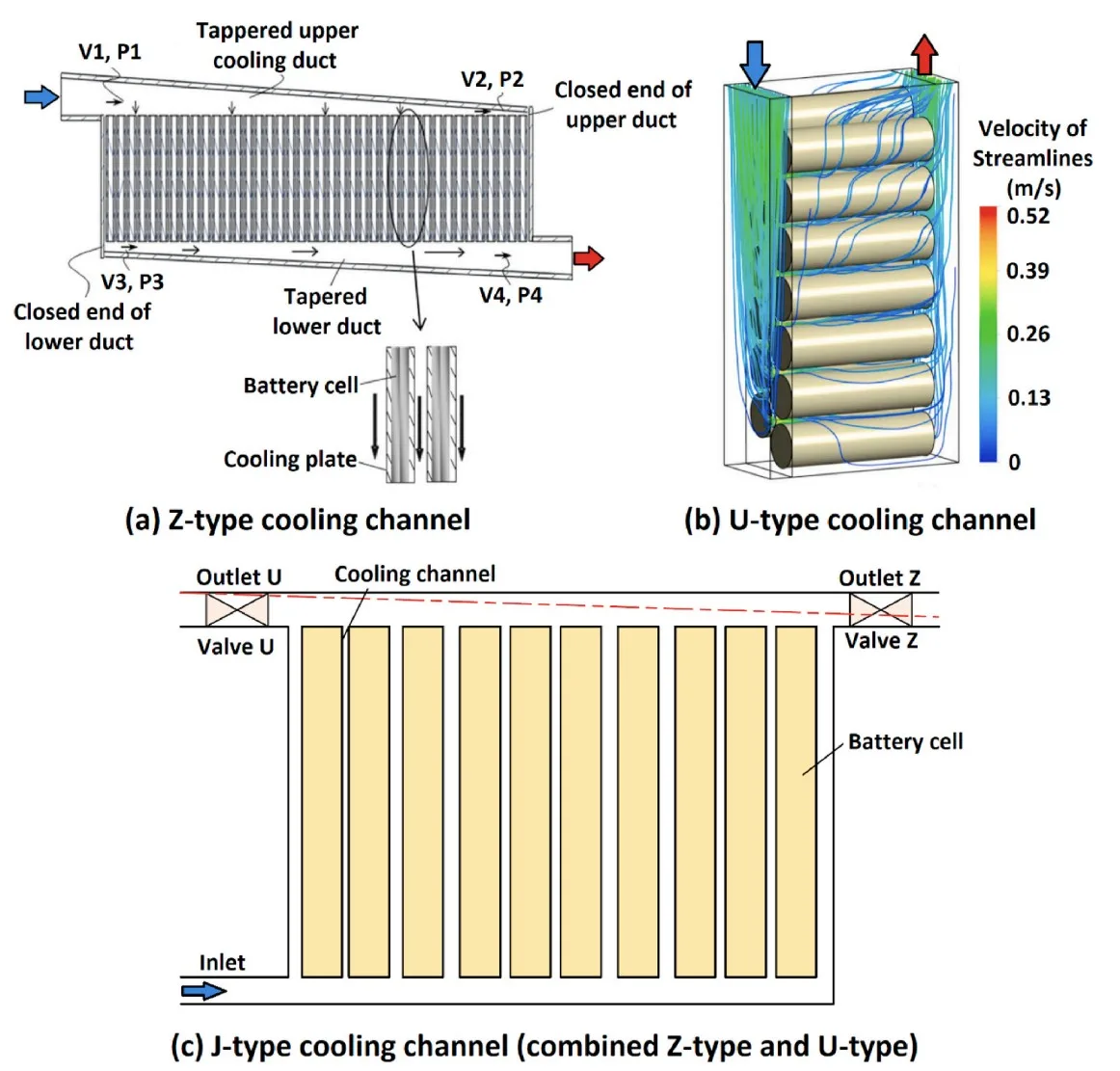
6. 結論と考察:
結論では、空冷式BTMSの長所と短所が要約されていると考えられます。空冷式BTMSは費用対効果が高く、実装が容易ですが、極端な動作条件(高温環境、高Cレートの充放電など)では十分な冷却性能が得られない可能性があります。考察セクションでは、冷却性能、コスト、複雑さ、重量のトレードオフについて検討し、空冷式BTMSのパフォーマンスを向上させるための潜在的な戦略(液冷やPCMなどの他の技術とのハイブリッドアプローチの検討など)を提案していると考えられます。空冷式BTMSの課題と限界に対処するためのさらなる研究の必要性が強調されているでしょう。
7. 今後の研究:
今後の研究の方向性としては、以下の点が考えられます。
- さまざまな動作条件下でバッテリーパック内の温度分布を正確に予測する、より高度な熱モデルの開発。
- 実世界での動作条件下での提案された設計改良の実験的検証。
- パフォーマンスと安全性を向上させるハイブリッドシステムを作成するために、空冷と他のBTMS技術の統合を検討すること。
- 先進的なバッテリー化学と設計との空冷式BTMSの適合性の調査。
8. 参考文献:
- [1] L.H. Martinez, Post industrial revolution human activity and climate change: why the United States must implement mandatory limits on industrial greenhouse gas emmissions, J. Land Use Environ. Law (2005) 403–421.
- [2] O. Edenhofer, Climate Change 2014: Mitigation of Climate Change, Cambridge University Press, 2015.
- [3] IEA. CO2 emissions by sector. https://www.iea.org/data-and-statistics?countr y=WORLD&fuel=CO2%20emissions&indicator=CO2BySector (accessed 3 December, 2020).
- [4] W. Yuan, H.C. Frey, T. Wei, N. Rastogi, S. VanderGriend, D. Miller, L. Mattison, Comparison of real-world vehicle fuel use and tailpipe emissions for gasolineethanol fuel blends, Fuel 249 (2019) 352–364.
- [5] X. Sun, Z. Li, X. Wang, C. Li, Technology development of electric vehicles: a review, Energies 13 (1) (2020) 90.
- [6] O. Gippner, D. Torney, Shifting policy priorities in EU-China energy relations: implications for Chinese energy investments in Europe, Energy Pol. 101 (2017) 649–658.
- [7] M. Debert, G. Colin, G. Bloch, Y. Chamaillard, An observer looks at the cell temperature in automotive battery packs, Contr. Eng. Pract. 21 (8) (2013) 1035–1042.
- [8] P. Wolfram, N. Lutsey, Electric Vehicles: Literature Review of Technology Costs and Carbon Emissions, The International Council on Clean Transportation, Washington, DC, USA, 2016, pp. 1–23.
- [9] G. Berckmans, M. Messagie, J. Smekens, N. Omar, L. Vanhaverbeke, J. Van Mierlo, Cost projection of state of the art lithium-ion batteries for electric vehicles up to 2030, Energies 10 (9) (2017) 1314.
- [10] F.V. Conte, Battery and battery management for hybrid electric vehicles: a review, E I Elektrotechnik Inf. 123 (10) (2006) 424–431.
- [11] Z.M. Salameh, M.A. Casacca, W.A. Lynch, A mathematical model for lead-acid batteries, IEEE Trans. Energy Convers. 7 (1) (1992) 93–98.
- [12] S. Ovshinsky, M. Fetcenko, J. Ross, A nickel metal hydride battery for electric vehicles, Science 260 (5105) (1993) 176–181.
- [13] S. Yoon, J. Lee, T. Hyeon, S.M. Oh, Electric double-layer capacitor performance of a new mesoporous carbon, J. Electrochem. Soc. 147 (7) (2000) 2507–2512.
- [14] L.H. Saw, Y. Ye, A.A.O. Tay, Integration issues of lithium-ion battery into electric vehicles battery pack, J. Clean. Prod. 113 (2016) 1032–1045.
- [15] L.I.R. Batteries, Technical Handbook, disponible 25, 2013, pp. 27–29, http://car di.igeofcu.unam.mx/techdocs/PowerSonic_batteries.pdf.
- [16] M.S. Whittingham, Materials challenges facing electrical energy storage, MRS Bull. 33 (4) (2008) 411–419.
- [17] G. Pistoia, Lithium-ion Batteries: Advances and Applications, Newnes, 2013.
- [18] N. Nitta, F. Wu, J.T. Lee, G. Yushin, Li-ion battery materials: present and future, Mater. Today 18 (5) (2015) 252–264.
- [19] A. Mahmoudzadeh Andwari, A. Pesiridis, S. Rajoo, R. Martinez-Botas, V. Esfahanian, A review of Battery Electric Vehicle technology and readiness levels, Renew. Sustain. Energy Rev. 78 (2017) 414–430.
- [20] N. Sato, Thermal behavior analysis of lithium-ion batteries for electric and hybrid vehicles, J. Power Sources 99 (1–2) (2001) 70–77.
- [21] S. Al-Hallaj, J.R. Selman, Thermal modeling of secondary lithium batteries for electric vehicle/hybrid electric vehicle applications, J. Power Sources 110 (2) (2002) 341–348.
- [22] L. Fred. Tesla Model 3: exclusive first look at Tesla’s new battery pack architecture. Electrek. https://electrek.co/2017/08/24/tesla-model-3-exclusi ve-battery-pack-architecture/(accessed 31 December, 2019).
- [23] C. Luca. BMW plans different sized battery cells to preserve design of future models. Automotive News Europe. https://europe.autonews.com/article/ 20180418/ANE/180419811/bmw-plans-different-sized-battery-cells-to-preservedesign-of-future-models (accessed 31 December, 2019).
- [24] D. Phil. Honda e battery specs and platform revealed, 31,000 have ‘expressed interest’ in the electric car. Electrek. https://electrek.co/2019/06/13/honda-ebattery-platform/(accessed 31 December, 2019).
- [25] G. Auto. Emgrand EV, Fashionably eco-feiendly. http://global.geely.com/car /emgrand-ev-2/(accessed 31 December, 2019).
- [26] G. Renault. Zoe – the ultimate city car, designed for everyday practicality and convenience. https://group.renault.com/en/innovation-2/electric-vehicl e/(accessed 31 December, 2019).
- [27] B. Matt. Hyundai Kona electric 2019 review – Australia. Carsales. https://www.ca rsales.com.au/editorial/details/hyundai-kona-electric-2019-review-australia -117573/?&tracking=dsa&gclid=Cj0KCQiA0ZHwBRCRARIsAK0Tr-raGraRwr_h15 AjJfiyhWKNIOluj3Y6XhBUFQbaibgZvklKXUFF05jVYaAiEYEALw_wcB&gclsrc =aw.ds (accessed 31 December, 2019).
- [28] A. E. Paul. Exclusive: FCA has big news coming in electrification. The Detroit Bureau. https://www.thedetroitbureau.com/2019/06/exclusive-fca-has-big-ne ws-coming-in-electrification/(accessed 31 December, 2019).
- [29] Z. Rao, S. Wang, A review of power battery thermal energy management, Renew. Sustain. Energy Rev. 15 (9) (2011) 4554–4571.
- [30] Q. Wang, B. Jiang, B. Li, Y. Yan, A critical review of thermal management models and solutions of lithium-ion batteries for the development of pure electric vehicles, Renew. Sustain. Energy Rev. 64 (2016) 106–128.
- [31] Z. An, L. Jia, Y. Ding, C. Dang, X. Li, A review on lithium-ion power battery thermal management technologies and thermal safety, J. Therm. Sci. 26 (5) (2017) 391–412.
- [32] G. Xia, L. Cao, G. Bi, A review on battery thermal management in electric vehicle application, J. Power Sources 367 (2017) 90–105.
- [33] J. Kim, J. Oh, H. Lee, Review on battery thermal management system for electric vehicles, Appl. Therm. Eng. 149 (2019) 192–212.
- [34] H. Liu, Z. Wei, W. He, J. Zhao, Thermal issues about Li-ion batteries and recent progress in battery thermal management systems: a review, Energy Convers. Manag. 150 (2017) 304–330.
- [35] W. Wu, S. Wang, W. Wu, K. Chen, S. Hong, Y. Lai, A critical review of battery thermal performance and liquid based battery thermal management, Energy Convers. Manag. 182 (2019) 262–281.
- [36] A.H. Akinlabi, D. Solyali, Configuration, design, and optimization of air-cooled battery thermal management system for electric vehicles: a review, Renew. Sustain. Energy Rev. 125 (2020) 109815.
- [37] M.S. Islam, D.J. Driscoll, C.A. Fisher, P.R. Slater, Atomic-scale investigation of defects, dopants, and lithium transport in the LiFePO4 olivine-type battery material, Chem. Mater. 17 (20) (2005) 5085–5092.
- [38] C. Daniel, D. Mohanty, J. Li, D.L. Wood, Cathode Materials Review, 2014.
- [39] S.-L. Wu, W. Zhang, X. Song, A.K. Shukla, G. Liu, V. Battaglia, V. Srinivasan, High rate capability of Li (Ni1/3Mn1/3Co1/3) O2 electrode for Li-ion batteries, J. Electrochem. Soc. 159 (4) (2012) A438–A444.
- [40] H.-J. Noh, S. Youn, C.S. Yoon, Y.-K. Sun, Comparison of the structural and electrochemical properties of layered Li
- [NixCoyMnz] O2 (x= 1/3, 0.5, 0.6, 0.7, 0.8 and 0.85) cathode material for lithium-ion batteries, J. Power Sources 233 (2013) 121–130.
- [41] P. Kalyani, N. Kalaiselvi, Various aspects of LiNiO2chemistry: a review, Sci. Technol. Adv. Mater. 6 (6) (2016) 689–703.
- [42] T.-F. Yi, Y.-R. Zhu, X.-D. Zhu, J. Shu, C.-B. Yue, A.-N. Zhou, A review of recent developments in the surface modification of LiMn2O4 as cathode material of power lithium-ion battery, Ionics 15 (6) (2009) 779–784.
- [43] C. Forgez, D.V. Do, G. Friedrich, M. Morcrette, C. Delacourt, Thermal modeling of a cylindrical LiFePO4/graphite lithium-ion battery, J. Power Sources 195 (9) (2010) 2961–2968.
- [44] W.-J. Zhang, Structure and performance of LiFePO4 cathode materials: a review, J. Power Sources 196 (6) (2011) 2962–2970.
- [45] C. Taubert, ¨ M. Fleischhammer, M. Wohlfahrt-Mehrens, U. Wietelmann, T. Buhrmester, LiBOB as electrolyte salt or additive for lithium-ion batteries based on LiNi0. 8Co0. 15Al0. 05O2/graphite, J. Electrochem. Soc. 157 (6) (2010) A721–A728.
- [46] D. Farhat, F. Ghamouss, J. Maibach, K. Edstrom, ¨ D. Lemordant, Adiponitrile–lithium bis (trimethylsulfonyl) imide solutions as Alkyl carbonatefree electrolytes for Li4Ti5O12 (LTO)/LiNi1/3Co1/3Mn1/3O2 (NMC) Li-ion batteries, ChemPhysChem 18 (10) (2017) 1333–1344.
- [47] Q. Fan, S. Yang, J. Liu, H. Liu, K. Lin, R. Liu, C. Hong, L. Liu, Y. Chen, K. An, Mixed-conducting interlayer boosting the electrochemical performance of Ni-rich layered oxide cathode materials for lithium ion batteries, J. Power Sources 421 (2019) 91–99.
- [48] Y. Jin, H. Yu, Y. Gao, X. He, T.A. White, X. Liang, Li4Ti5O12 coated with ultrathin aluminum-doped zinc oxide films as an anode material for lithium-ion batteries, J. Power Sources 436 (2019), 226859.
- [49] M. Han, J. Yu, Subnanoscopically and homogeneously dispersed SiOx/C composite spheres for high-performance lithium ion battery anodes, J. Power Sources 414 (2019) 435–443.
- [50] Y. Xiang, W. Zhang, B. Chen, Z. Jin, H. Zhang, P. Zhao, G. Cao, Q. Meng, NanoLi4Ti5O12 particles in-situ deposited on compact holey-graphene framework for high volumetric power capability of lithium ion battery anode, J. Power Sources 447 (2020) 227372.
- [51] M.E. Sotomayor, C. de La Torre-Gamarra, B. Levenfeld, J.-Y. Sanchez, A. Varez, G.-T. Kim, A. Varzi, S. Passerini, Ultra-thick battery electrodes for high gravimetric and volumetric energy density Li-ion batteries, J. Power Sources 437 (2019) 226923.
- [52] D. Li, L. Chen, L. Chen, Q. Sun, M. Zhu, Y. Zhang, Y. Liu, Z. Liang, P. Si, J. Lou, Potassium gluconate-derived N/S Co-doped carbon nanosheets as superior electrode materials for supercapacitors and sodium-ion batteries, J. Power Sources 414 (2019) 308–316.
- [53] X. Shi, Q. Sun, B. Boateng, Y. Niu, Y. Han, W. Lv, W. He, A quasi-solid composite separator with high ductility for safe and high-performance lithium-ion batteries, J. Power Sources 414 (2019) 225–232.
- [54] J. Oh, H. Jo, H. Lee, H.-T. Kim, Y.M. Lee, M.-H. Ryou, Polydopamine-treated three-dimensional carbon fiber-coated separator for achieving high-performance lithium metal batteries, J. Power Sources 430 (2019) 130–136.
- [55] J.R. Nair, F. Colo, ` A. Kazzazi, M. Moreno, D. Bresser, R. Lin, F. Bella, G. Meligrana, S. Fantini, E. Simonetti, Room temperature ionic liquid (RTIL)- based electrolyte cocktails for safe, high working potential Li-based polymer batteries, J. Power Sources 412 (2019) 398–407.
- [56] B. Peng, Y. Li, J. Gao, F. Zhang, J. Li, G. Zhang, High energy K-ion batteries based on P3-Type K0⋅ 5MnO2 hollow submicrosphere cathode, J. Power Sources 437 (2019), 226913.
- [57] Y. Wang, P. Cui, W. Zhu, Z. Feng, M.-J. Vigeant, H. Demers, A. Guerfi, K. Zaghib, Enhancing the electrochemical performance of an O3–NaCrO2 cathode in sodium-ion batteries by cation substitution, J. Power Sources 435 (2019), 226760.
- [58] J. Ming, J. Guo, C. Xia, W. Wang, H.N. Alshareef, Zinc-ion batteries: materials, mechanisms, and applications, Mater. Sci. Eng. R Rep. 135 (2019) 58–84.
- [59] S. Chen, Y. Zhang, H. Geng, Y. Yang, X. Rui, C.C. Li, Zinc ions pillared vanadate cathodes by chemical pre-intercalation towards long cycling life and lowtemperature zinc ion batteries, J. Power Sources 441 (2019), 227192.
- [60] H. Xiao. Battery thermal management in electric vehicles. ANSYS. https ://support.ansys.com/staticassets/ANSYS/staticassets/resourcelibrary/whitepa per/wp-battery-thermal-management.pdf (accessed 19 January, 2020).
- [61] D. Bernardi, E. Pawlikowski, J. Newman, A general energy balance for battery systems, J. Electrochem. Soc. 132 (1) (1985) 5.
- [62] Q. Norman. Industry developments in thermal management of electric vehicle batteries. Advanced Thermal Solutions. https://www.qats.com/cms/category/ battery-cooling/(accessed 19 January, 2020).
- [63] J. Gate, Rechargeable Batteries Applications Handbook, Newnes, Newton, 1998.
- [64] K. Jalkanen, T. Aho, K. Vuorilehto, Entropy change effects on the thermal behavior of a LiFePO4/graphite lithium-ion cell at different states of charge, J. Power Sources 243 (2013) 354–360.
- [65] X. Feng, H.B. Gooi, S.X. Chen, An improved lithium-ion battery model with temperature prediction considering entropy, in: 2012 3rd IEEE PES Innovative Smart Grid Technologies Europe (ISGT Europe), IEEE, 2012, pp. 1–8.
- [66] J. Newman, K.E. Thomas-Alyea, Electrochemical Systems, John Wiley & Sons, 2012.
- [67] Y. Xie, X.-j. He, X.-s. Hu, W. Li, Y.-j. Zhang, B. Liu, Y.-t. Sun, An improved resistance-based thermal model for a pouch lithium-ion battery considering heat generation of posts, Appl. Therm. Eng. 164 (2020), 114455.
- [68] Y. Kobayashi, H. Miyashiro, K. Kumai, K. Takei, T. Iwahori, I. Uchida, Precise electrochemical calorimetry of LiCoO2/graphite lithium-ion cell: understanding thermal behavior and estimation of degradation mechanism, J. Electrochem. Soc. 149 (8) (2002) A978.
- [69] K. Chen, G. Unsworth, X. Li, Measurements of heat generation in prismatic Li-ion batteries, J. Power Sources 261 (2014) 28–37.
- [70] S. Drake, M. Martin, D. Wetz, J. Ostanek, S. Miller, J. Heinzel, A. Jain, Heat generation rate measurement in a Li-ion cell at large C-rates through temperature and heat flux measurements, J. Power Sources 285 (2015) 266–273.
- [71] J. Zhang, J. Huang, Z. Li, B. Wu, Z. Nie, Y. Sun, F. An, N. Wu, Comparison and validation of methods for estimating heat generation rate of large-format lithiumion batteries, J. Therm. Anal. Calorim. 117 (1) (2014) 447–461.
- [72] B. Wu, Z. Li, J. Zhang, Thermal design for the pouch-type large-format lithiumion batteries: I. Thermo-electrical modeling and origins of temperature nonuniformity, J. Electrochem. Soc. 162 (1) (2014) A181.
- [73] E. Schuster, C. Ziebert, A. Melcher, M. Rohde, H.J. Seifert, Thermal behavior and electrochemical heat generation in a commercial 40 Ah lithium ion pouch cell, J. Power Sources 286 (2015) 580–589.
- [74] Y. Saito, M. Shikano, H. Kobayashi, Heat generation behavior during charging and discharging of lithium-ion batteries after long-time storage, J. Power Sources 244 (2013) 294–299.
- [75] Y. Ye, L.H. Saw, Y. Shi, A.A. Tay, Numerical analyses on optimizing a heat pipe thermal management system for lithium-ion batteries during fast charging, Appl. Therm. Eng. 86 (2015) 281–291.
- [76] X. Xu, R. He, Review on the heat dissipation performance of battery pack with different structures and operation conditions, Renew. Sustain. Energy Rev. 29 (2014) 301–315.
- [77] C. Wang, G. Zhang, L. Meng, X. Li, W. Situ, Y. Lv, M. Rao, Liquid cooling based on thermal silica plate for battery thermal management system, Int. J. Energy Res. 41 (15) (2017) 2468–2479.
- [78] L. Sheng, L. Su, H. Zhang, Y. Fang, H. Xu, W. Ye, An improved calorimetric method for characterizations of the specific heat and the heat generation rate in a prismatic lithium ion battery cell, Energy Convers. Manag. 180 (2019) 724–732.
- [79] Z.H. Wu Qingyu, Junwei Li, Calibrated calorimetry for measuring the specific heat capacityand heat generation rate of lithium-ion battery, Automot. Eng. 42 (1) (2020) 59–65.
- [80] P. Ramadass, B. Haran, R. White, B.N. Popov, Capacity fade of Sony 18650 cells cycled at elevated temperatures: Part I. Cycling performance, J. Power Sources 112 (2) (2002) 606–613.
- [81] P. Ramadass, B. Haran, R. White, B.N. Popov, Capacity fade of Sony 18650 cells cycled at elevated temperatures: Part II. Capacity fade analysis, J. Power Sources 112 (2) (2002) 614–620.
- [82] A. Szumanowski, Y. Chang, Battery management system based on battery nonlinear dynamics modeling, IEEE Trans. Veh. Technol. 57 (3) (2008) 1425–1432.
- [83] J. Belt, V. Utgikar, I. Bloom, Calendar and PHEV cycle life aging of high-energy, lithium-ion cells containing blended spinel and layered-oxide cathodes, J. Power Sources 196 (23) (2011) 10213–10221.
- [84] D. Aurbach, Y. Talyosef, B. Markovsky, E. Markevich, E. Zinigrad, L. Asraf, J. S. Gnanaraj, H.-J. Kim, Design of electrolyte solutions for Li and Li-ion batteries: a review, Electrochim. Acta 50 (2–3) (2004) 247–254.
- [85] R. Zhao, J. Liu, J. Gu, The effects of electrode thickness on the electrochemical and thermal characteristics of lithium ion battery, Appl. Energy 139 (2015) 220–229.
- [86] J. Li, E. Murphy, J. Winnick, P. Kohl, Studies on the cycle life of commercial lithium ion batteries during rapid charge–discharge cycling, J. Power Sources 102 (1–2) (2001) 294–301.
- [87] Q. Zhang, R.E. White, Capacity fade analysis of a lithium ion cell, J. Power Sources 179 (2) (2008) 793–798.
- [88] Y. Kitagawa, K. Kato, M. Fukui, Analysis and experimentation for effective cooling of Li-ion batteries, Procedia Technology 18 (2014) 63–67.
- [89] W. Waag, S. K¨ abitz, D.U. Sauer, Experimental investigation of the lithium-ion battery impedance characteristic at various conditions and aging states and its influence on the application, Appl. Energy 102 (2013) 885–897.
- [90] S. Saxena, C. Hendricks, M. Pecht, Cycle life testing and modeling of graphite/ LiCoO2 cells under different state of charge ranges, J. Power Sources 327 (2016) 394–400.
- [91] L. Su, J. Zhang, C. Wang, Y. Zhang, Z. Li, Y. Song, T. Jin, Z. Ma, Identifying main factors of capacity fading in lithium ion cells using orthogonal design of experiments, Appl. Energy 163 (2016) 201–210.
- [92] M.M. Kabir, D.E. Demirocak, Degradation mechanisms in Li-ion batteries: a stateof-the-art review, Int. J. Energy Res. 41 (14) (2017) 1963–1986.
- [93] D. Li, D.L. Danilov, H.J. Bergveld, R.-A. Eichel, P.H. Notten, Understanding battery aging mechanisms, in: Future Lithium-Ion Batteries, 2019, pp. 220–250.
- [94] K. Smith, E. Wood, S. Santhanagopalan, G. Kim, A. Pesaran, Advanced models and controls for prediction and extension of battery lifetime, in: Advanced Automotive Battery Conference, Atlanta, GA, 2014, pp. 4–6.
- [95] F. Leng, C.M. Tan, M. Pecht, Effect of temperature on the aging rate of Li ion battery operating above room temperature, Sci. Rep. 5 (2015) 12967.
- [96] H. Gabrisch, Y. Ozawa, R. Yazami, Crystal structure studies of thermally aged LiCoO2 and LiMn2O4 cathodes, Electrochim. Acta 52 (4) (2006) 1499–1506.
- [97] X. Zhou, J. Huang, Z. Pan, M. Ouyang, Impedance characterization of lithium-ion batteries aging under high-temperature cycling: importance of electrolyte-phase diffusion, J. Power Sources 426 (2019) 216–222.
- [98] Z. Tang, S. Wang, Z. Liu, J. Cheng, Numerical analysis of temperature uniformity of a liquid cooling battery module composed of heat-conducting blocks with gradient contact surface angles, Appl. Therm. Eng. 178 (2020) 115509.
- [99] P. Ni, X. Wang, Temperature field and temperature difference of a battery package for a hybrid car, Case Stud. Therm. Eng. (2020) 100646.
- [100] A.A. Pesaran, Battery thermal models for hybrid vehicle simulations, J. Power Sources 110 (2) (2002) 377–382.
- [101] C. Park, A.K. Jaura, Dynamic Thermal Model of Li-Ion Battery for Predictive Behavior in Hybrid and Fuel Cell Vehicles, SAE Technical Paper, 0148-7191, 2003.
- [102] Z. Rao, S. Wang, G. Zhang, Simulation and experiment of thermal energy management with phase change material for ageing LiFePO4 power battery, Energy Convers. Manag. 52 (12) (2011) 3408–3414.
- [103] H. Park, A design of air flow configuration for cooling lithium ion battery in hybrid electric vehicles, J. Power Sources 239 (2013) 30–36.
- [104] L. Panasonic, Co. Lithium Ion NCR18650PF, 2016, June, p. 31
- [Online]. Available, https://www.omnitron.cz/download/datasheet/NCR-18650PF.pdf. (Accessed December 2019).
- [105] X.-H. Yang, S.-C. Tan, J. Liu, Thermal management of Li-ion battery with liquid metal 117, Energy Conversion and Management, 2016, pp. 577–585.
- [106] A. Schmidt, A. Smith, H. Ehrenberg, Power capability and cyclic aging of commercial, high power lithium ion battery cells with respect to different cell designs, J. Power Sources 425 (2019) 27–38.
- [107] Q. Wang, P. Ping, X. Zhao, G. Chu, J. Sun, C. Chen, Thermal runaway caused fire and explosion of lithium ion battery, J. Power Sources 208 (2012) 210–224.
- [108] S. Arora, W. Shen, A. Kapoor, Review of mechanical design and strategic placement technique of a robust battery pack for electric vehicles, Renew. Sustain. Energy Rev. 60 (2016) 1319–1331.
- [109] V. Ruiz, A. Pfrang, A. Kriston, N. Omar, P. Van den Bossche, L. Boon-Brett, A review of international abuse testing standards and regulations for lithium ion batteries in electric and hybrid electric vehicles, Renew. Sustain. Energy Rev. 81 (2018) 1427–1452.
- [110] A.W. Golubkov, D. Fuchs, J. Wagner, H. Wiltsche, C. Stangl, G. Fauler, G. Voitic, A. Thaler, V. Hacker, Thermal-runaway experiments on consumer Li-ion batteries with metal-oxide and olivin-type cathodes, RSC Adv. 4 (7) (2014) 3633–3642.
- [111] H.M. Barkholtz, Y. Preger, S. Ivanov, J. Langendorf, L. Torres-Castro, J. Lamb, B. Chalamala, S.R. Ferreira, Multi-scale thermal stability study of commercial lithium-ion batteries as a function of cathode chemistry and state-of-charge, J. Power Sources 435 (2019) 226777.
- [112] S. Hossain, Y.-K. Kim, Y. Saleh, R. Loutfy, Overcharge studies of carbon fiber composite-based lithium-ion cells, J. Power Sources 161 (1) (2006) 640–647.
- [113] E. Roth, D. Doughty, D. Pile, Effects of separator breakdown on abuse response of 18650 Li-ion cells, J. Power Sources 174 (2) (2007) 579–583.
- [114] C.L. Campion, W. Li, B.L. Lucht, Thermal decomposition of LiPF6-based electrolytes for lithium-ion batteries, J. Electrochem. Soc. 152 (12) (2005) A2327–A2334.
- [115] N.P. Lebedeva, F.D. Persio, T. Kosmidou, D. Dams, A. Pfrang, A. Kersys, L. BoonBrett, Amount of free liquid electrolyte in commercial large format prismatic Liion battery cells, J. Electrochem. Soc. 166 (4) (2019) A779–A786.
- [116] V. Peter, GM Recalls Volts to Fix Fire Risk, The Comeback of the American Car, CNN Money, 2012.
- [117] R. Charles, Tesla Dodges Full Investigation after Fiery Crash, CNN Money, Hongkong, 2013.
- [118] A. Pfrang, A. Kriston, V. Ruiz, N. Lebedeva, F. Di Persio, Safety of rechargeable energy storage systems with a focus on Li-ion technology, in: Emerging Nanotechnologies in Rechargeable Energy Storage Systems, Elsevier, 2017, pp. 253–290.
- [119] X. Feng, S. Zheng, D. Ren, X. He, L. Wang, H. Cui, X. Liu, C. Jin, F. Zhang, C. Xu, Investigating the thermal runaway mechanisms of lithium-ion batteries based on thermal analysis database, Appl. Energy 246 (2019) 53–64.
- [120] X. Feng, D. Ren, X. He, M. Ouyang, Mitigating Thermal Runaway of Lithium-Ion Batteries, Joule, 2020.
- [121] D.H. Doughty, E.P. Roth, A general discussion of Li ion battery safety, The Electrochemical Society Interface 21 (2) (2012) 37–44.
- [122] Y.S. Choi, D.M. Kang, Prediction of thermal behaviors of an air-cooled lithium-ion battery system for hybrid electric vehicles, J. Power Sources 270 (2014) 273–280.
- [123] Q. Wang, X. Zhao, J. Ye, Q. Sun, P. Ping, J. Sun, Thermal response of lithium-ion battery during charging and discharging under adiabatic conditions, J. Therm. Anal. Calorim. 124 (1) (2016) 417–428.
- [124] A.A. Pesaran, Battery thermal management in EV and HEVs: issues and solutions, Battery Man. 43 (5) (2001) 34–49.
- [125] K. Kelly, J. Rugh, A. Pesaran, Improving battery thermal management using design for six sigma process, in: For Presentation at the 20 th Electric Vehicle Symposium, Long Beach, CA November 15-18, 2003, 2003.
- [126] Y. Ye, Y. Shi, N. Cai, J. Lee, X. He, Electro-thermal modeling and experimental validation for lithium ion battery, J. Power Sources 199 (2012) 227–238.
- [127] L. Lu, X. Han, J. Li, J. Hua, M. Ouyang, A review on the key issues for lithium-ion battery management in electric vehicles, J. Power Sources 226 (2013) 272–288.
- [128] J. Jaguemont, L. Boulon, Y. Dub´e, A comprehensive review of lithium-ion batteries used in hybrid and electric vehicles at cold temperatures, Appl. Energy 164 (2016) 99–114.
- [129] R. Koyama, Y. Arai, Y. Yamauchi, S. Takeya, F. Endo, A. Hotta, R. Ohmura, Thermophysical properties of trimethylolethane (TME) hydrate as phase change material for cooling lithium-ion battery in electric vehicle, J. Power Sources 427 (2019) 70–76.
- [130] J. Chen, S. Kang, E. Jiaqiang, Z. Huang, K. Wei, B. Zhang, H. Zhu, Y. Deng, F. Zhang, G. Liao, Effects of different phase change material thermal management strategies on the cooling performance of the power lithium ion batteries: a review, J. Power Sources 442 (2019) 227228.
- [131] R. Zhao, S. Zhang, J. Liu, J. Gu, A review of thermal performance improving methods of lithium ion battery: electrode modification and thermal management system, J. Power Sources 299 (2015) 557–577.
- [132] D. Chen, J. Jiang, G.-H. Kim, C. Yang, A. Pesaran, Comparison of different cooling methods for lithium ion battery cells, Appl. Therm. Eng. 94 (2016) 846–854.
- [133] R. Kizilel, R. Sabbah, J.R. Selman, S. Al-Hallaj, An alternative cooling system to enhance the safety of Li-ion battery packs, J. Power Sources 194 (2) (2009) 1105–1112.
- [134] M.H. Westbrook, M. Westbrook, The Electric Car: Development and Future of Battery, Hybrid and Fuel-Cell Cars, Iet 38, 2001.
- [135] M. Arasu, Q. Ahmed, G. Rizzoni, Optimizing Battery Cooling System for a Range Extended Electric Truck, SAE Technical Paper, 0148-7191, 2019.
- [136] S. Park, D. Jung, Battery cell arrangement and heat transfer fluid effects on the parasitic power consumption and the cell temperature distribution in a hybrid electric vehicle, J. Power Sources 227 (2013) 191–198.
- [137] M.R. Giuliano, A.K. Prasad, S.G. Advani, Experimental study of an air-cooled thermal management system for high capacity lithium–titanate batteries, J. Power Sources 216 (2012) 345–352.
- [138] A. Pesaran, G. Kim, Battery Thermal Management System Design Modeling, National Renewable Energy Lab.(NREL), Golden, CO (United States), 2006.
- [139] K.J. Kelly, M. Mihalic, M. Zolot, Battery usage and thermal performance of the Toyota Prius and Honda Insight during chassis dynamometer testing, in: Seventeenth Annual Battery Conference on Applications and Advances. Proceedings of Conference (Cat. No. 02TH8576), IEEE, 2002, pp. 247–252.
- [140] A. Pesaran, M. Keyser, S. Burch, An Approach for Designing Thermal Management Systems for Electric and Hybrid Vehicle Battery Packs, National Renewable Energy Laboratory, Golden, CO (US), 1999.
- [141] Volkswagen E-up! Air cooled battery, featured in ViaVision magazine. My electric car forums. https://www.myelectriccarforums.com/volkswagen-e-up-air-cooledbattery-featured-in-viavision-magazine/(accessed 19 January, 2020).
- [142] L. Andrew. Volkswagen’s EV racecar just broke records during this year’s Pikes Peak Hill Climb. The Verge. https://www.theverge.com/2018/6/24/1 7078544/volkswagen-ev-race-car-pikes-peak-hill-climb-record (accessed 19 January, 2020).
- [143] D. G. Marcus. Lexus’ first-ever electric vehicle is the UX300e. Autoindustriya. htt ps://www.autoindustriya.com/auto-industry-news/lexus-first-ever-electric-veh icle-is-the-ux300e.html (accessed 20 January, 2020).
- [144] L. Eric. Here’s why nissan employs active air cooling in e-NV200 battery pack. InsideEVs. https://insideevs.com/news/322347/heres-why-nissan-employs-act ive-air cooling-in-e-nv200-battery-pack/(accessed 20 January, 2020).
- [145] P. Rodgers, V. Eveloy, M.G. Pecht, Limits of air cooling: status and challenges, in: Semiconductor Thermal Measurement and Management IEEE Twenty First Annual IEEE Symposium, IEEE, 2005, pp. 116–124.
- [146] R. Jilte, R. Kumar, Numerical investigation on cooling performance of Li-ion battery thermal management system at high galvanostatic discharge, Eng. Sci. Technology. Int. J. 21 (5) (2018) 957–969.
- [147] C.-W. Park, A.K. Jaura, Thermal Analysis of Cooling System in Hybrid Electric Vehicles, SAE Technical Paper, 0148-7191, 2002.
- [148] T. Wang, K.J. Tseng, J. Zhao, Development of efficient air cooling strategies for lithium-ion battery module based on empirical heat source model, Appl. Therm. Eng. 90 (2015) 521–529.
- [149] D.C. Erb, S. Kumar, S.E. Sarma, E. Carlson, Size matters: why cell size is vital for minimizing cost of air cooling in battery packs, in: 2015 IEEE Transportation Electrification Conference and Expo (ITEC), IEEE, 2015, pp. 1–6.
- [150] T. Wang, K.J. Tseng, J. Zhao, Z. Wei, Thermal investigation of lithium-ion battery module with different cell arrangement structures and forced air cooling strategies, Appl. Energy 134 (2014) 229–238.
- [151] D. Kang, P.-Y. Lee, K. Yoo, J. Kim, Internal thermal network model-based inner temperature distribution of high-power lithium-ion battery packs with different shapes for thermal management, J. Energy Stor. vol. 27, 2020.
- [152] Y. Zhang, X. Song, C. Ma, D. Hao, Y. Chen, Effects of the structure arrangement and spacing on the thermal characteristics of Li-ion battery pack at various discharge rates, Appl. Therm. Eng. 165 (2020).
- [153] N. Yang, X. Zhang, G. Li, D. Hua, Assessment of the forced air cooling performance for cylindrical lithium-ion battery packs: a comparative analysis between aligned and staggered cell arrangements, Appl. Therm. Eng. 80 (2015) 55–65.
- [154] Y. Fan, Y. Bao, C. Ling, Y. Chu, X. Tan, S. Yang, Experimental study on the thermal management performance of air cooling for high energy density cylindrical lithium-ion batteries, Appl. Therm. Eng. 155 (2019) 96–109.
- [155] M. Ye, Y. Xu, Y. Huangfu, The structure optimization of lithium-ion battery pack based on fluid-solid conjugate thermodynamic analysis, Energy Procedia 152 (2018) 643–648.
- [156] K. Chen, S. Wang, M. Song, L. Chen, Configuration optimization of battery pack in parallel air-cooled battery thermal management system using an optimization strategy, Appl. Therm. Eng. 123 (2017) 177–186.
- [157] T. Yang, N. Yang, X. Zhang, G. Li, Investigation of the thermal performance of axial-flow air cooling for the lithium-ion battery pack, Int. J. Therm. Sci. 108 (2016) 132–144.
- [158] X. Yu, Z. Lu, L. Zhang, L. Wei, X. Cui, L. Jin, Experimental study on transient thermal characteristics of stagger-arranged lithium-ion battery pack with air cooling strategy, Int. J. Heat Mass Tran. 143 (2019) 118576.
- [159] B. Severino, F. Gana, R. Palma-Behnke, P.A. Est´evez, W.R. Calderon-Mu ´ noz, ˜ M. E. Orchard, J. Reyes, M. Cort´es, Multi-objective optimal design of lithium-ion battery packs based on evolutionary algorithms, J. Power Sources 267 (2014) 288–299.
- [160] W. Li, M. Xiao, X. Peng, A. Garg, L. Gao, A surrogate thermal modeling and parametric optimization of battery pack with air cooling for EVs, Appl. Therm. Eng. 147 (2019) 90–100.
- [161] L. Fan, J.M. Khodadadi, A.A. Pesaran, A parametric study on thermal management of an air-cooled lithium-ion battery module for plug-in hybrid electric vehicles, J. Power Sources 238 (2013) 301–312.
- [162] J. Xun, R. Liu, K. Jiao, Numerical and analytical modeling of lithium ion battery thermal behaviors with different cooling designs, J. Power Sources 233 (2013) 47–61.
- [163] X.M. Xu, R. He, Research on the heat dissipation performance of battery pack based on forced air cooling, J. Power Sources 240 (2013) 33–41.
- [164] H. Sun, R. Dixon, Development of cooling strategy for an air cooled lithium-ion battery pack, J. Power Sources 272 (2014) 404–414.
- [165] K. Chen, M. Song, W. Wei, S. Wang, Structure optimization of parallel air-cooled battery thermal management system with U-type flow for cooling efficiency improvement, Energy 145 (2018) 603–613.
- [166] Z. Lu, X. Yu, L. Wei, Y. Qiu, L. Zhang, X. Meng, L. Jin, Parametric study of forced air cooling strategy for lithium-ion battery pack with staggered arrangement, Appl. Therm. Eng. 136 (2018) 28–40.
- [167] Y. Liu, J. Zhang, Design a J-type air-based battery thermal management system through surrogate-based optimization, Appl. Energy 252 (2019).
- [168] K. Yu, X. Yang, Y. Cheng, C. Li, Thermal analysis and two-directional air flow thermal management for lithium-ion battery pack, J. Power Sources 270 (2014) 193–200.
- [169] H. Fathabadi, A novel design including cooling media for Lithium-ion batteries pack used in hybrid and electric vehicles, J. Power Sources 245 (2014) 495–500.
- [170] H. Zhou, F. Zhou, L. Xu, J. Kong, QingxinYang, Thermal performance of cylindrical Lithium-ion battery thermal management system based on air distribution pipe, Int. J. Heat Mass Tran. 131 (2019) 984–998.
- [171] Z. Lu, X.Z. Meng, L.C. Wei, W.Y. Hu, L.Y. Zhang, L.W. Jin, Thermal management of densely-packed EV battery with forced air cooling strategies, Energy Procedia 88 (2016) 682–688.
- [172] H. Wang, L. Ma, Thermal management of a large prismatic battery pack based on reciprocating flow and active control, Int. J. Heat Mass Tran. 115 (2017) 296–303.
- [173] X. Na, H. Kang, T. Wang, Y. Wang, Reverse layered air flow for Li-ion battery thermal management, Appl. Therm. Eng. 143 (2018) 257–262.
- [174] M. Soltani, G. Berckmans, J. Jaguemont, J. Ronsmans, S. Kakihara, O. Hegazy, J. Van Mierlo, N. Omar, Three dimensional thermal model development and validation for lithium-ion capacitor module including air cooling system, Appl. Therm. Eng. 153 (2019) 264–274.
- [175] J. Zhao, Z. Rao, Y. Li, Thermal performance of mini-channel liquid cooled cylinder based battery thermal management for cylindrical lithium-ion power battery, Energy Convers. Manag. 103 (2015) 157–165.
- [176] K. Chen, W. Wu, F. Yuan, L. Chen, S. Wang, Cooling efficiency improvement of air-cooled battery thermal management system through designing the flow pattern, Energy 167 (2019) 781–790.
- [177] K. Chen, Y. Chen, Y. She, M. Song, S. Wang, L. Chen, Construction of effective symmetrical air-cooled system for battery thermal management, Appl. Therm. Eng. 166 (2020) 114679.
- [178] Y.-W. Wang, J.-M. Jiang, Y.-H. Chung, W.-C. Chen, C.-M. Shu, Forced-air cooling system for large-scale lithium-ion battery modules during charge and discharge processes, J. Therm. Anal. Calorim. 135 (5) (2018) 2891–2901.
- [179] J. E, M. Yue, J. Chen, H. Zhu, Y. Deng, Y. Zhu, F. Zhang, M. Wen, B. Zhang, S. Kang, Effects of the different air cooling strategies on cooling performance of a lithium-ion battery module with baffle, Appl. Therm. Eng. 144 (2018) 231–241.
- [180] S. Shahid, M. Agelin-Chaab, Experimental and numerical studies on air cooling and temperature uniformity in a battery pack, Int. J. Energy Res. 42 (6) (2018) 2246–2262.
- [181] S. Shahid, M. Agelin-Chaab, Analysis of cooling effectiveness and temperature uniformity in a battery pack for cylindrical batteries, Energies 10 (8) (2017) 1157.
- [182] K. Chen, Z. Li, Y. Chen, S. Long, J. Hou, M. Song, S. Wang, Design of parallel aircooled battery thermal management system through numerical study, Energies 10 (10) (2017).
- [183] S. Hong, X. Zhang, K. Chen, S. Wang, Design of flow configuration for parallel aircooled battery thermal management system with secondary vent, Int. J. Heat Mass Tran. 116 (2018) 1204–1212.
- [184] C. Zhu, X. Li, L. Song, L. Xiang, Development of a theoretically based thermal model for lithium ion battery pack, J. Power Sources 223 (2013) 155–164.
- [185] L.H. Saw, Y. Ye, M.C. Yew, W.T. Chong, M.K. Yew, T.C. Ng, Computational fluid dynamics simulation on open cell aluminium foams for Li-ion battery cooling system, Appl. Energy 204 (2017) 1489–1499.
- [186] X. Li, F. He, G. Zhang, Q. Huang, D. Zhou, Experiment and simulation for pouch battery with silica cooling plates and copper mesh based air cooling thermal management system, Appl. Therm. Eng. 146 (2019) 866–880.
- [187] S.K. Mohammadian, S.M. Rassoulinejad-Mousavi, Y. Zhang, Thermal management improvement of an air-cooled high-power lithium-ion battery by embedding metal foam, J. Power Sources 296 (2015) 305–313.
- [188] S.K. Mohammadian, Y. Zhang, Cumulative effects of using pin fin heat sink and porous metal foam on thermal management of lithium-ion batteries, Appl. Therm. Eng. 118 (2017) 375–384.
- [189] R.D. Jilte, R. Kumar, M.H. Ahmadi, L. Chen, Battery thermal management system employing phase change material with cell-to-cell air cooling, Appl. Therm. Eng. 161 (2019).
- [190] P. Qin, M. Liao, D. Zhang, Y. Liu, J. Sun, Q. Wang, Experimental and numerical study on a novel hybrid battery thermal management system integrated forced-air convection and phase change material, Energy Convers. Manag. 195 (2019) 1371–1381.
- [191] M. Mehrabi-Kermani, E. Houshfar, M. Ashjaee, A novel hybrid thermal management for Li-ion batteries using phase change materials embedded in copper foams combined with forced-air convection, Int. J. Therm. Sci. 141 (2019) 47–61.
- [192] W. Luo, F. He, Q. Huang, X. Li, G. Zhang, Z. Zhong, Experimental investigation on thermal performance of silica cooling plate-aluminate thermal plate-coupled forced convection-based pouch battery thermal management system, Int. J. Energy Res. 43 (13) (2019) 7604–7613.
- [193] L.H. Saw, H.M. Poon, H.S. Thiam, Z. Cai, W.T. Chong, N.A. Pambudi, Y.J. King, Novel thermal management system using mist cooling for lithium-ion battery packs, Appl. Energy 223 (2018) 146–158.
- [194] A.M. Sefidan, A. Sojoudi, S.C. Saha, Nanofluid-based cooling of cylindrical lithium-ion battery packs employing forced air flow, Int. J. Therm. Sci. 117 (2017) 44–58.
- [195] R. Zhao, J. Liu, J. Gu, L. Zhai, F. Ma, Experimental study of a direct evaporative cooling approach for Li-ion battery thermal management, Int. J. Energy Res. (2020).
- [196] S.K. Mohammadian, Y. Zhang, Thermal management optimization of an aircooled Li-ion battery module using pin-fin heat sinks for hybrid electric vehicles, J. Power Sources 273 (2015) 431–439.
- [197] D. Dan, C. Yao, Y. Zhang, H. Zhang, Z. Zeng, X. Xu, Dynamic thermal behavior of micro heat pipe array-air cooling battery thermal management system based on thermal network model, Appl. Therm. Eng. 162 (2019).
- [198] T. Han, B. Khalighi, E.C. Yen, S. Kaushik, Li-ion battery pack thermal management: liquid versus air cooling, J. Therm. Sci. Eng. Appl. 11 (2) (2019).
- [199] W. Li, A. Jishnu, A. Garg, M. Xiao, X. Peng, L. Gao, Heat transfer efficiency enhancement of lithium-ion battery packs by using novel design of herringbone fins, J. Electrochem. Energy Convers. Stor. 17 (2) (2020).
- [200] Y. Wei, M. Agelin-Chaab, Development and experimental analysis of a hybrid cooling concept for electric vehicle battery packs, J. Energy Stor. 25 (2019) 100906.
- [201] Y. Zhao, Y. Patel, T. Zhang, G.J. Offer, Modeling the effects of thermal gradients induced by tab and surface cooling on lithium ion cell performance, J. Electrochem. Soc. 165 (13) (2018) A3169.
- [202] I.A. Hunt, Y. Zhao, Y. Patel, G. Offer, Surface cooling causes accelerated degradation compared to tab cooling for lithium-ion pouch cells, J. Electrochem. Soc. 163 (9) (2016) A1846.
- [203] O. Dondelewski, T.S. O’Connor, Y. Zhao, I.A. Hunt, A. Holland, A. Hales, G. J. Offer, Y. Patel, The role of cell geometry when selecting tab or surface cooling to minimise cell degradation, eTransportation 5 (2020) 100073.
- [204] S. Li, N. Kirkaldy, C. Zhang, K. Gopalakrishnan, T. Amietszajew, L.B. Diaz, J. V. Barreras, M. Shams, X. Hua, Y. Patel, Optimal cell tab design and cooling strategy for cylindrical lithium-ion batteries, J. Power Sources 492 (2021), 229594
- [205] H. Heimes, A. Kampker, A. Mohsseni, F. Maltoni, J. Biederbeck, Cell tab cooling system for battery life extension, in: 2019 18th IEEE Intersociety Conference on Thermal and Thermomechanical Phenomena in Electronic Systems (ITherm), IEEE, 2019, pp. 1125–1133.
- [206] M.S. Patil, J.-H. Seo, M.-Y. Lee, A novel dielectric fluid immersion cooling technology for Li-ion battery thermal management, Energy Convers. Manag. 229 (2021) 113715.
- [207] J.-C. Jang, S.-H. Rhi, Battery thermal management system of future electric vehicles with loop thermosyphon, in: US-Korea Conference on Science, Technology, and Entrepreneurship, UKC), 2010.
- [208] P. Gupta, S. Vardhan, Optimizing OEE, productivity and production cost for improving sales volume in an automobile industry through TPM: a case study, Int. J. Prod. Res. 54 (10) (2016) 2976–2988.
- [209] A. Brooker, M. Thornton, J. Rugh, Technology Improvement Pathways to CostEffective Vehicle Electrification, 2010.
- [210] R.J. Orsato, P. Wells, U-turn: the rise and demise of the automobile industry, J. Clean. Prod. 15 (11–12) (2007) 994–1006.
- [211] K. Kisu, S. Kim, H. Oguchi, N. Toyama, S.-i. Orimo, Interfacial stability between LiBH4-based complex hydride solid electrolytes and Li metal anode for all-solidstate Li batteries, J. Power Sources 436 (2019) 226821.
- [212] Y. Zhang, W. Lu, L. Cong, J. Liu, L. Sun, A. Mauger, C.M. Julien, H. Xie, J. Liu, Cross-linking network based on Poly (ethylene oxide): solid polymer electrolyte for room temperature lithium battery, J. Power Sources 420 (2019) 63–72.
- [213] B. Zhang, L. Chen, J. Hu, Y. Liu, Y. Liu, Q. Feng, G. Zhu, L.-Z. Fan, Solid-state lithium metal batteries enabled with high loading composite cathode materials and ceramic-based composite electrolytes, J. Power Sources 442 (2019) 227230.
- [214] H. Nguyen, A. Banerjee, X. Wang, D. Tan, E.A. Wu, J.-M. Doux, R. Stephens, G. Verbist, Y.S. Meng, Single-step synthesis of highly conductive Na3PS4 solid electrolyte for sodium all solid-state batteries, J. Power Sources 435 (2019), 126623
Copyright and Acknowledgements:
This summary is based on the paper "A novel automated heat-pipe cooling device for high-power LEDs" by Chengdi Xiao et al.
https://doi.org/10.1016/j.jpowsour.2021.230001
Copyright © 2025 CASTMAN. All rights reserved.