本論文概要は、NADCA Die Casting Congress & Expositionで発表された論文「Influence of Die Temperature in High Pressure Die Casting of Thin-Walled Components」に基づいています。
1. 概要:
- タイトル: 薄肉部品の高圧ダイカストにおける金型温度の影響 (薄肉部品のコウアツダイカストにおけるカナガタオンドノエイキョウ)
- 著者: M. Wessén, L. Näslund
- 発表年: 2015年
- 発表ジャーナル/学会: NADCA Die Casting Congress & Exposition
- キーワード: 高圧ダイカスト (コウアツダイカスト, HPDC), 金型温度 (カナガタオンド), 薄肉部品 (ハクニクブヒン), 充填 (ジュウテン), 凝固 (ギョウコ), 機械的特性 (キカイテキトクセイ), 組織 (ソシキ)
![Figure 3.1: The process chain of high pressure die casting. (adapted from [62])](https://castman.co.kr/wp-content/uploads/image-238-png.webp)
2. 研究背景:
- 研究トピックの社会的/学術的背景: 高圧ダイカスト (HPDC) は、複雑な形状のニアネットシェイプ部品を大量生産するための広く利用されている製造プロセスです。自動車産業などの軽量化要求の高まりにより、材料消費量を削減し効率を向上させるために、より薄肉の鋳造部品の需要が高まっています。しかし、薄肉部品の鋳造は、急速な熱伝達と欠陥の可能性のためにHPDCにおいて課題を伴います。
- 既存研究の限界: 論文では、金型温度はHPDCにおける重要なパラメータである一方で、薄肉部品の鋳造に対するその影響は十分に理解されていないと述べています。既存の研究は、急速な凝固や、それが充填、組織、機械的特性に与える影響など、薄肉部に関連する特定の問題に十分に対処していない可能性があります。
- 研究の必要性: 高品質な薄肉HPDC部品を製造するためには、金型温度の影響をより深く理解することが不可欠です。この研究は、薄肉形状に対するHPDCプロセスを最適化するために必要であり、構造的完全性を維持しながら、より軽量で効率的な部品の製造を可能にすることを目的としています。
3. 研究目的と研究課題:
- 研究目的: 本研究の目的は、薄肉部品の高圧ダイカスト (HPDC) プロセスにおける金型温度の影響を調査することです。この研究は、金型温度の変化が、充填プロセス、凝固挙動、組織形成、そして最終的には鋳造部品の機械的特性にどのように影響するかを理解することを目的としています。
- 主な研究課題:
- 金型温度は、薄肉HPDC部品における充填パターンと充填時間にどのように影響するか?
- 金型温度は、薄肉鋳造品の凝固プロセスと結果として得られる組織にどのような影響を与えるか?
- 金型温度は、薄肉HPDC部品の機械的特性、特に引張強度と伸びにどのように影響するか?
- 研究仮説: 本研究では、金型温度が薄肉HPDC部品の充填、凝固、組織、および機械的特性に大きな影響を与えると仮説を立てています。具体的には、金型温度が高いほど、充填が改善され、気孔率が減少し、組織が微細化され、機械的特性が向上すると予想されますが、ある時点を超えると悪影響が生じる可能性があります。
4. 研究方法
- 研究デザイン: 本研究では、工業用高圧ダイカスト設備を用いた実験的アプローチを採用しています。この研究では、金型温度の影響を分離するために、他のプロセスパラメータを一定に保ちながら金型温度を変化させます。
- データ収集方法:
- プロセスモニタリング: 鋳造プロセス中の金型温度と圧力を監視するためにセンサーが使用されました。
- 目視検査: 鋳造品を目視検査し、表面欠陥や未充填がないか確認しました。
- X線コンピュータ断層撮影 (CT): CTスキャンを使用して、鋳造品内の気孔率分布を分析しました。
- 顕微鏡観察: 光学顕微鏡を使用して、鋳造品の組織を観察しました。
- 機械試験: 引張試験を実施して、鋳造品の機械的特性(引張強度と伸び)を評価しました。
- 分析方法:
- 定性的分析: 目視検査と組織分析により、鋳造品質と組織の特徴に関する定性的なデータが得られました。
- 定量的分析: CTスキャンにより、気孔率体積分率に関する定量的なデータが得られました。引張試験により、機械的特性に関する定量的なデータが得られました。統計分析はおそらく、金型温度と測定変数の関係を分析するために使用されました。
- 研究対象と範囲: 本研究は、アルミニウム合金製の薄肉部品の高圧ダイカストに焦点を当てています。特定の合金と部品形状は論文で詳しく説明されています。範囲は、工業用HPDC設備を使用して、特定の薄肉部品設計における特定の範囲内の金型温度の影響を調査することに限定されています。
5. 主な研究結果:
- 主な研究結果:
- 充填: 「金型温度が高いほど、薄肉部の充填が改善された。」「充填時間は、金型温度の上昇とともにわずかに減少した。」
- 凝固: 「金型温度の上昇は、凝固速度の低下につながった。」
- 組織: 「組織は金型温度の影響を受けた。金型温度が高いほど、結晶粒径が大きく、共晶シリコン量が増加した粗大な組織になった。」「気孔率レベルは、金型温度の上昇とともに一定の点まで減少し、その後、気孔率がわずかに再び増加した。」
- 機械的特性: 「引張強度と伸びは金型温度の影響を受けた。機械的特性を最大化するための最適な金型温度範囲が見つかった。」「引張強度と伸びはどちらも、最初は金型温度とともに増加し、最大値に達した後、最も高い金型温度で減少した。」
- 統計的/定性的分析結果:
- 論文には、充填時間、気孔率、引張強度、伸びの傾向を金型温度の関数として示す図が示されています。これらの図は、観察された定量的な関係を視覚的に表しています。
- 異なる金型温度での組織の変化を定性的に示す顕微鏡写真が提示されています。
- CTスキャン画像は、異なる金型温度で製造された鋳造品の気孔率分布を示しています。
- データ解釈:
- 金型温度の上昇は、溶融金属の粘度を下げ、凝固を遅らせることによって充填を改善し、溶融金属が薄肉部に効果的に流れ込むことを可能にします。
- 金型温度が高いほど凝固が遅くなるため、組織が粗大になります。
- 金型温度の上昇に伴う気孔率の初期の減少は、充填の改善と空気の巻き込みの減少に起因すると考えられます。最も高い温度での気孔率のわずかな増加は、高温での溶融金属へのガス溶解度の増加または他の要因による可能性があります。
- 機械的特性に最適な金型温度は、充填と組織の微細化(低温)と、粗大な組織だがより良い充填(高温)のバランスを表しています。
- 図のリスト:
- 図1:実験装置の概略図。
- 図2:金型温度の関数としての充填時間。
- 図3:金型温度の関数としての気孔率体積分率。
- 図4:異なる金型温度での組織写真。
- 図5:金型温度の関数としての引張強度。
- 図6:金型温度の関数としての伸び。
![Figure 3.4: As-cast microstructure of HPDC processed primary alloy: (a) SEM image showing
primary α-Al and eutectic Al-Si (adapted from [77]), and (b - d) Synchrotron X-ray tomography
images of α-Fe intermetallics showing their 3D morphology of (b) polyhedral, (c) fne compact,
and (d) Chinese script (adapted from [78]).](https://castman.co.kr/wp-content/uploads/image-239-1024x580.webp)
![Figure 3.5: SEM images on the fracture surface of HPDC processed primary alloy showing: (a) gas pores, and (b) shrinkage pore. (adapted from [79])](https://castman.co.kr/wp-content/uploads/image-240-png.webp)
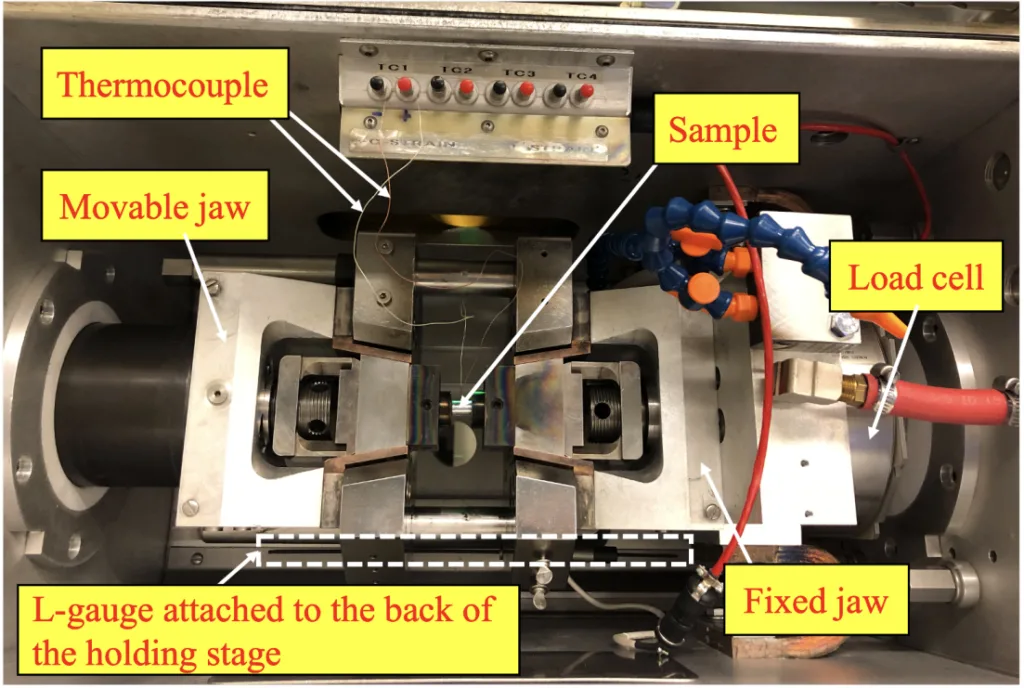
![Figure 4.2: (a) Schematic presentation of SHPB setup used to perform high strain rate compression tests (adapted from [102]), and (b) actual SHPB setup showing the pressure bars, sample,
thermocouple, ceramic wool, induction coil and water bucket.](https://castman.co.kr/wp-content/uploads/image-242-1024x649.webp)
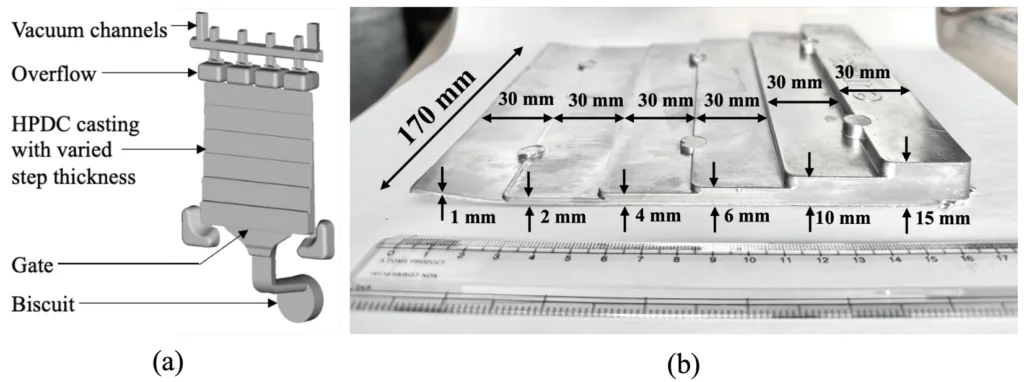
6. 結論と考察:
- 主な結果の要約: 本研究は、金型温度が薄肉部品のHPDCに大きな影響を与えることを示しています。金型温度を上げると、充填が改善され、気孔率が一定の点まで減少し、組織が粗大になり、機械的特性に影響を与えます。充填、組織、および機械的性能のバランスをとるための最適な金型温度範囲が存在します。
- 研究の学術的意義: 本研究は、薄肉HPDCにおける金型温度とプロセス結果の間の複雑な相互作用のより良い理解に貢献します。特定の状況下で金型温度が充填、凝固、組織、および機械的特性に影響を与えるメカニズムに関する貴重な実験データと洞察を提供します。
- 実用的な意義: この知見は、薄肉部品のHPDCプロセスを最適化するための実用的な意義を持っています。ダイカストメーカーは、この情報を使用して適切な金型温度を選択し、鋳造品質を向上させ、欠陥を減らし、薄肉HPDC部品の機械的性能を向上させることができます。最適な金型温度範囲の特定は、プロセスの最適化にとって特に価値があります。
- 研究の限界: 本研究は、特定のアルミニウム合金、部品形状、および金型温度範囲に限定されています。結果は、他の合金、部品設計、またはプロセス条件に直接転用できない場合があります。より広範囲の条件下で金型温度の影響を調査するには、さらなる研究が必要です。
7. 今後のフォローアップ研究:
- 今後のフォローアップ研究の方向性:
- 他のアルミニウム合金や異なる薄肉部品形状に対する金型温度の影響を調査する。
- プロセスの最適化をより包括的に理解するために、金型温度と、射出速度や圧力などの他のプロセスパラメータの組み合わせの効果を探る。
- 金型温度と組織の関係をさらに解明するために、金属間化合物相や気孔率の形態の特性評価など、より詳細な組織分析を実施する。
- 疲労強度や衝撃靭性など、他の機械的特性に対する金型温度の影響を調査する。
- プロセス設計と最適化を支援するために、金型温度の影響を組み込んだ薄肉部品のHPDCプロセスをシミュレーションするための数値モデルを開発する。
- さらなる探求が必要な分野: 論文では、最も高い金型温度での気孔率のわずかな増加の背後にあるメカニズムと、異なる金型温度での組織の進化の詳細な調査について、さらなる探求が必要であることを示唆しています。
8. 参考文献:
- [1] V. Singh. “Non-ferrous metals and alloys”. In: Physical Metallurgy, pp. 679–688.
- [2] Aerospace Specifcation Metals Inc. 2018.
- [3] R. Muraca and J. Whittick, eds. Materials Data Handbook: Aluminum Alloy 7075. 2nd. San Carlos, California: Western Applied Research & Development, 1972.
- [4] “Heat Treating of Aluminum Alloys”. In: ASM Handbook. Vol. 4. 1991, pp. 841– 879.
- [5] N. Mahathaninwong, T. Plookphol, J. Wannasin, and S. Wisutmethangoon. “T6 heat treatment of rheocasting 7075 Al alloy”. In: Materials Science and Engineering A 532 (2012), pp. 91–99.
- [6] X.-L. Zou, H. Yan, and X.-H. Chen. “Evolution of second phases and mechanical properties of 7075 Al alloy processed by solution heat treatment”. In: Transactions of Nonferrous Metals Society of China (English Edition) 27.10 (2017), pp. 2146– 2155.
- [7] Q. Liu, S. Chen, R. Gu, W. Wang, and X. Wei. “Efect of Heat Treatment Conditions on Mechanical Properties and Precipitates in Sheet Metal Hot Stamping of 7075 Aluminum Alloy”. In: Journal of Materials Engineering and Performance 27.9 (Sept. 2018), pp. 4423–4436.
- [8] L. F. Mondolfo, N. A. Gjostein, and D. W. Levinson. “Structural Changes During the Aging in An Al-Mg-Zn Alloy”. In: JOM 8.10 (Oct. 1956), pp. 1378–1385.
- [9] L. F. Mondolfo. “Structure of the aluminium: magnesium: zinc alloys”. In: Metallurgical Reviews 16.1 (Jan. 1971), pp. 95–124.
- [10] J. Embury and R. Nicholson. “The nucleation of precipitates: The system Al-ZnMg”. In: Acta Metallurgica 13.4 (Apr. 1965), pp. 403–417.
- [11] H. L¨ofer, I. Kovacs, and J. Lendvai. “Decomposition processes in AI-Zn-Mg alloys”. In: Journal of Materials Science 18 (1983), pp. 2215–2240.
- [12] J. G. Kaufman. “Understanding the Aluminum Temper Designation System”. In: Introduction to Aluminium Alloys and Tempers. ASM International, 2000. Chap. 4, pp. 39–76.
- [13] R. Goswami, S. Lynch, N. J. H. Holroyd, S. P. Knight, and R. L. Holtz. “Evolution of Grain Boundary Precipitates in Al 7075 Upon Aging and Correlation with Stress Corrosion Cracking Behavior”. In: Metallurgical and Materials Transactions A 44.3 (Mar. 2013), pp. 1268–1278.
- [14] T. Hu, K. Ma, T. Topping, J. Schoenung, and E. Lavernia. “Precipitation phenomena in an ultrafne-grained Al alloy”. In: Acta Materialia 61.6 (Apr. 2013), pp. 2163–2178.
- [15] R. DeIasi and P. N. Adler. “Calorimetric studies of 7000 series aluminum alloys: I. Matrix precipitate characterization of 7075”. In: Metallurgical Transactions A 8.7 (July 1977), pp. 1177–1183.
- [16] J. I. Rojas and D. Crespo. “Dynamic microstructural evolution of an Al–Zn–Mg–Cu alloy (7075) during continuous heating and the infuence on the viscoelastic response”. In: Materials Characterization 134 (Dec. 2017), pp. 319–328.
- [17] T. Engdahl, V. Hansen, P. Warren, and K. Stiller. “Investigation of fne scale precipitates in Al–Zn–Mg alloys after various heat treatments”. In: Materials Science and Engineering: A 327.1 (Apr. 2002), pp. 59–64.
- [18] J. K. Park and A. J. Ardell. “Microstructures of the commercial 7075 Al alloy in the T651 and T7 tempers”. In: Metallurgical Transactions A 14.10 (Oct. 1983), pp. 1957–1965.
- [19] J. Park and A. Ardell. “Precipitation at grain boundaries in the commercial alloy Al 7075”. In: Acta Metallurgica 34.12 (Dec. 1986), pp. 2399–2409.
- [20] J. Park and A. Ardell. “Precipitate microstructure of peak-aged 7075 Al”. In: Scripta Metallurgica 22.7 (Jan. 1988), pp. 1115–1119.
- [21] X. Li, V. Hansen, J. GjØnnes, and L. Wallenberg. “HREM study and structure modeling of the η’ phase, the hardening precipitates in commercial Al–Zn–Mg alloys”. In: Acta Materialia 47.9 (July 1999), pp. 2651–2659.
- [22] J. Auld and S. Mck. Cousland. “The transition phase η’ in Al-Zn-Mg alloys”. In: Scripta Metallurgica 5.9 (Sept. 1971), pp. 765–769.
- [23] J. Gjønnes and C. Simensen. “An electron microscope investigation of the microstructure in an aluminium-zinc-magnesium alloy”. In: Acta Metallurgica 18.8 (Aug. 1970), pp. 881–890.
- [24] W. Zhang, X. Chen, B. Zhuo, P. Li, and L. He. “Efect of strain rate and temperature on dynamic mechanical behavior and microstructure evolution of ultra-high strength aluminum alloy”. In: Materials Science and Engineering: A 730.June (July 2018), pp. 336–344.
- [25] J.-F. Li, Z.-W. Peng, C.-X. Li, Z.-Q. Jia, W.-J. Chen, and Z.-Q. Zheng. “Mechanical properties, corrosion behaviors and microstructures of 7075 aluminium alloy with various aging treatments”. In: Transactions of Nonferrous Metals Society of China 18.4 (Aug. 2008), pp. 755–762.
- [26] P.-L. Mao, B. Yu, Z. Liu, F. Wang, and Y. Ju. “Mechanical properties and electronic structures of MgCu2, Mg2Ca and MgZn2 Laves phases by frst principles calculations”. In: Transactions of Nonferrous Metals Society of China 24.9 (Sept. 2014), pp. 2920–2929.
- [27] P. A. Rometsch, Y. Zhang, and S. Knight. “Heat treatment of 7xxx series aluminium alloys—Some recent developments”. In: Transactions of Nonferrous Metals Society of China 24.7 (July 2014), pp. 2003–2017.
- [28] R. Ayer, J. Y. Koo, J. W. Steeds, and B. K. Park. “Microanalytical study of the heterogeneous phases in commercial Al-Zn-Mg-Cu alloys”. In: Metallurgical Transactions A 16.11 (Nov. 1985), pp. 1925–1936.
- [29] R. Zhang, G. Zhao, H. Yu, G. Shuai, L. Wu, C. Jin, L. He, and C. Kang. “High Temperature Deformation and Microstructure Evolution Behaviours of 7075 Cast Aluminum Alloy under Multi-pass Loading”. In: IOP Conference Series: Materials Science and Engineering 611.1 (Oct. 2019), p. 012003.
- [30] X. Fan, D. Jiang, Q. Meng, and L. Zhong. “The microstructural evolution of an Al–Zn–Mg–Cu alloy during homogenization”. In: Materials Letters 60.12 (June 2006), pp. 1475–1479.
- [31] E. Starke and J. Staley. “Application of modern aluminium alloys to aircraft”. In: Fundamentals of Aluminium Metallurgy. Vol. 32. 95. Elsevier, 2011, pp. 747–783.
- [32] F. Andreatta, H. Terryn, and J. H. de Wit. “Efect of solution heat treatment on galvanic coupling between intermetallics and matrix in AA7075-T6”. In: Corrosion Science 45.8 (2003), pp. 1733–1746.
- [33] M. Gao, C. R. Feng, and R. P. Wei. “An analytical electron microscopy study of constituent particles in commercial 7075-T6 and 2024-T3 alloys”. In: Metallurgical and Materials Transactions A 29.4 (Apr. 1998), pp. 1145–1151.
- [34] UACJ Corporation. Aluminium Forged Products for Aerospace Applications.
- [35] T. Dursun and C. Soutis. “Recent developments in advanced aircraft aluminium alloys”. In: Materials & Design (1980-2015) 56 (Apr. 2014), pp. 862–871.
- [36] S. I. Wright, M. M. Nowell, and D. P. Field. “A review of strain analysis using Electron Backscatter Difraction”. In: Microscopy and Microanalysis 17 (2011), pp. 316–329.
- [37] R. Doherty, D. Hughes, F. Humphreys, J. Jonas, D. Jensen, M. Kassner, W. King, T. McNelley, H. McQueen, and A. Rollett. “Current issues in recrystallization: a review”. In: Materials Science and Engineering: A 238.2 (Nov. 1997), pp. 219–274.
- [38] K. Huang and R. E. Log´e. “A review of dynamic recrystallization phenomena in metallic materials”. In: Materials and Design 111 (2016), pp. 548–574.
- [39] S. Gourdet and F. Montheillet. “An experimental study of the recrystallization mechanism during hot deformation of aluminium”. In: Materials Science and Engineering: A 283.1-2 (May 2000), pp. 274–288.
- [40] S. Gourdet and F. Montheillet. “A model of continuous dynamic recrystallization”. In: Acta Materialia 51.9 (2003), pp. 2685–2699.
- [41] A. Azimi, G. M. Owolabi, H. Fallahdoost, N. Kumar, and G. Warner. “High Strain Rate Behavior of Ultrafne Grained AA2519 Processed via Multi Axial Cryogenic Forging”. In: Metals 9.2 (Jan. 2019), p. 115.
- [42] Q. Xue, M. Meyers, and V. Nesterenko. “Self-organization of shear bands in titanium and Ti–6Al–4V alloy”. In: Acta Materialia 50.3 (Feb. 2002), pp. 575–596.
- [43] M. Taheri-Mandarjani, A. Zarei-Hanzaki, and H. Abedi. “Hot ductility behavior of an extruded 7075 aluminum alloy”. In: Materials Science and Engineering: A 637 (June 2015), pp. 107–122.
- [44] J. Lu, Y. Song, L. Hua, K. Zheng, and D. Dai. “Thermal deformation behavior and processing maps of 7075 aluminum alloy sheet based on isothermal uniaxial tensile tests”. In: Journal of Alloys and Compounds 767.July (Oct. 2018), pp. 856–869.
- [45] H. T. Jeong and W. J. Kim. “Comparison of hot deformation behavior characteristics between as-cast and extruded Al-Zn-Mg-Cu (7075) aluminum alloys with a similar grain size”. In: Materials 12.23 (2019), pp. 1–16.
- [46] S. Park and W. Kim. “Diference in the Hot Compressive Behavior and Processing Maps between the As-cast and Homogenized Al-Zn-Mg-Cu (7075) Alloys”. In: Journal of Materials Science & Technology 32.7 (July 2016), pp. 660–670.
- [47] M. Rajamuthamilselvan and S. Ramanathan. “Hot deformation behaviour of 7075 alloy”. In: Journal of Alloys and Compounds 509.3 (Jan. 2011), pp. 948–952.
- [48] Z.-C. Sun, J. H. Wang, Y. Wang, and H. Yang. “Hot Deformation Behavior and Fracture of As-Extruded 7075 Aluminum Alloy”. In: Advanced Materials Research 291-294 (July 2011), pp. 1051–1056.
- [49] M. Rokni, A. Zarei-Hanzaki, A. A. Roostaei, and H. Abedi. “An investigation into the hot deformation characteristics of 7075 aluminum alloy”. In: Materials & Design 32.4 (Apr. 2011), pp. 2339–2344.
- [50] W. Xiao, B. Wang, Y. Wu, and X. Yang. “Constitutive modeling of fow behavior and microstructure evolution of AA7075 in hot tensile deformation”. In: Materials Science and Engineering A 712 (2018), pp. 704–713.
- [51] Z.-C. Sun, L.-S. Zheng, and H. Yang. “Softening mechanism and microstructure evolution of as-extruded 7075 aluminum alloy during hot deformation”. In: Materials Characterization 90 (Apr. 2014), pp. 71–80.
- [52] X. Yang, H. Miura, and T. Sakai. “Continuous Dynamic Recrystallization in a Superplastic 7075 Aluminum Alloy”. In: MATERIALS TRANSACTIONS 43.10 (2002), pp. 2400–2407.
- [53] Z.-C. Sun, H. Wu, J. Cao, and Z. Yin. “Modeling of continuous dynamic recrystallization of Al-Zn-Cu-Mg alloy during hot deformation based on the internalstate-variable (ISV) method”. In: International Journal of Plasticity 106.November 2017 (July 2018), pp. 73–87.
- [54] G. T. Gray. “Classic Split-Hopkinson Pressure Bar Testing”. In: ASM Handbook. Ed. by H. Kuhn and D. Medlin. Vol. 8. 2000, pp. 462–476.
- [55] D. N. Zhang, Q. Q. Shangguan, C. J. Xie, and F. Liu. “A modifed Johnson-Cook model of dynamic tensile behaviors for 7075-T6 aluminum alloy”. In: Journal of Alloys and Compounds 619 (2015), pp. 186–194.
- [56] E. El-Magd and M. Abouridouane. “Characterization, modelling and simulation of deformation and fracture behaviour of the light-weight wrought alloys under high strain rate loading”. In: International Journal of Impact Engineering 32.5 (May 2006), pp. 741–758.
- [57] K. Senthil, M. Iqbal, P. Chandel, and N. Gupta. “Study of the constitutive behavior of 7075-T651 aluminum alloy”. In: International Journal of Impact Engineering 108 (Oct. 2017), pp. 171–190.
- [58] M. Sasso, A. Forcellese, M. Simoncini, D. Amodio, and E. Mancini. “High Strain Rate Behaviour of AA7075 Aluminum Alloy at Diferent Initial Temper States”. In: Key Engineering Materials 651-653 (July 2015), pp. 114–119.
- [59] W.-S. Lee, W.-C. Sue, C.-F. Lin, and C.-J. Wu. “The strain rate and temperature dependence of the dynamic impact properties of 7075 aluminum alloy”. In: Journal of Materials Processing Technology 100.1-3 (Apr. 2000), pp. 116–122.
- [60] W.-S. Lee and C.-R. Lin. “Deformation behavior and microstructural evolution of 7075-T6 aluminum alloy at cryogenic temperatures”. In: Cryogenics 79 (Oct. 2016), pp. 26–34.
- [61] T. Rahmaan, P. Zhou, C. Butcher, and M. J. Worswick. “Strain rate and thermal softening efects in shear testing of AA7075-T6 sheet”. In: EPJ Web of Conferences 183 (Sept. 2018). Ed. by E. Buzaud, A. Cosculluela, H. Couque, and E. Cadoni, p. 02037.
- [62] F. Bonollo, N. Gramegna, and G. Timelli. “High-Pressure Die-Casting: Contradictions and Challenges”. In: JOM 67.5 (May 2015), pp. 901–908.
- [63] B. M. Reddy and T. Nallusamy. “Degassing of Aluminum Metals and Its Alloys in Non-ferrous Foundry”. In: Springer Proceedings in Materials. Vol. 5. April. Springer Singapore, 2021, pp. 637–644.
- [64] J. G. Kaufman and E. L. Rooy. “Aluminum Casting Alloys”. In: Aluminum Alloy Castings: Properties, Processes, and Applications. ASM International, Nov. 2004, pp. 7–20.
- [65] T. O. Mbuya, B. O. Odera, and S. P. Ng’ang’a. “Infuence of iron on castability and properties of aluminium silicon alloys: literature review”. In: International Journal of Cast Metals Research 16.5 (Nov. 2003), pp. 451–465.
- [66] L. Wang, M. Makhlouf, and D. Apelian. “Aluminium die casting alloys: alloy composition, microstructure, and properties-performance relationships”. In: International Materials Reviews 40.6 (Jan. 1995), pp. 221–238.
- [67] L. Zhang, J. Gao, L. N. W. Damoah, and D. G. Robertson. “Removal of Iron From Aluminum: A Review”. In: Mineral Processing and Extractive Metallurgy Review 33.2 (Mar. 2012), pp. 99–157.
- [68] G. Gustafsson, T. Thorvaldsson, and G. L. Dunlop. “The infuence of Fe and Cr on the microstructure of cast Al-Si-Mg alloys”. In: Metallurgical Transactions A 17.1 (Jan. 1986), pp. 45–52.
- [69] P. Crepeau. Efect of Iron in Al-Si alloys: a critical review. 1995.
- [70] D. Apelian. “Aluminum Cast Alloys: Enabling Tools for Improved Performance”. In: World Wide Report. Wheeling, Illinois: North American Die Casting Association, 2009.
- [71] J. A. Taylor. “Iron-Containing Intermetallic Phases in Al-Si Based Casting Alloys”. In: Procedia Materials Science 1 (2012), pp. 19–33.
- [72] Rheinhfelden Alloys. Primary Aluminium Alloys for Pressure Die Casting. 2015.
- [73] L. A. Narayanan, F. H. Samuel, and J. E. Gruzleski. “Crystallization behavior of iron-containing intermetallic compounds in 319 aluminum alloy”. In: Metallurgical and Materials Transactions A 25.8 (Aug. 1994), pp. 1761–1773.
- [74] Trimet-05 data sheet. Trimal®-05: Die cast alloy for crash-relevant applications. 2008.
- [75] F. Liu, H. Zhao, R. Yang, and F. Sun. “Microstructure and Mechanical Properties of High Vacuum Die-Cast AlSiMgMn Alloys at as-Cast and T6-Treated Conditions”. In: Materials 12.13 (June 2019), p. 2065.
- [76] X. Jiao, Y. Liu, J. Wang, W. Liu, A. Wan, S. Wiesner, and S. Xiong. “The microstructure characteristics and fracture behavior of the polyhedral primary iron-rich phase and plate-shaped eutectic iron-rich phase in a high-pressure diecast AlSi10MnMg alloy”. In: Journal of Materials Science & Technology 140 (Mar. 2023), pp. 201–209.
- [77] X. Dong, X. Zhu, and S. Ji. “Efect of super vacuum assisted high pressure die casting on the repeatability of mechanical properties of Al-Si-Mg-Mn die-cast alloys”. In: Journal of Materials Processing Technology 266.August 2018 (Apr. 2019), pp. 105–113.
- [78] X. Jiao, C. Liu, Z. Guo, G. Tong, S. Ma, Y. Bi, Y. Zhang, and S. Xiong. “The characterization of Fe-rich phases in a high-pressure die cast hypoeutectic aluminumsilicon alloy”. In: Journal of Materials Science & Technology 51 (Aug. 2020), pp. 54–62.
- [79] R. Liu, J. Zheng, L. Godlewski, J. Zindel, M. Li, W. Li, and S. Huang. “Infuence of pore characteristics and eutectic particles on the tensile properties of Al–Si–Mn–Mg high pressure die casting alloy”. In: Materials Science and Engineering: A 783.March (May 2020), p. 139280.
- [80] X. Jiao, Y. Zhang, J. Wang, H. Nishat, Y. Liu, W. Liu, H. Chen, and S. Xiong. “Characterization of externally solidifed crystals in a high-pressure die-cast AlSi10MnMg alloy and their efect on porosities and mechanical properties”. In: Journal of Materials Processing Technology 298.July (Dec. 2021), p. 117299.
- [81] M. da Silva, J. Pujante, J. Hrabia-Wi´snios, B. Augustyn, D. Kapinos, M. Wgrzyn, and S. Boczkal. “Analysis of Inclusions and Impurities Present in Typical HPDC, Stamping and Extrusion Alloys Produced with Diferent Scrap Levels”. In: Metals 14.6 (May 2024), p. 626.
- [82] E. Cinkilic, M. Moodispaw, J. Zhang, J. Miao, and A. A. Luo. “A New Recycled Al–Si–Mg Alloy for Sustainable Structural Die Casting Applications”. In: Metallurgical and Materials Transactions A 53.8 (Aug. 2022), pp. 2861–2873.
- [83] D. B¨osch, S. Pogatscher, M. Hummel, W. Fragner, P. J. Uggowitzer, M. G¨oken, and H. W. H¨oppel. “Secondary Al-Si-Mg High-pressure Die Casting Alloys with Enhanced Ductility”. In: Metallurgical and Materials Transactions A 46.3 (Mar. 2015), pp. 1035–1045.
- [84] R. Lumley. “The Development of High Strength and Ductility in High-Pressure Die-Cast Al-Si-Mg Alloys from Secondary Sources”. In: JOM 71.1 (Jan. 2019), pp. 382–390.
- [85] A. Niklas, A. Baquedano, S. Orden, E. Nogu`es, M. Da Silva, and A. I. Fern´andezCalvo. “Microstructure and Mechanical Properties of a New Secondary AlSi10MnMg(Fe) Alloy for Ductile High Pressure Die Casting Parts for the Automotive Industry”. In: Key Engineering Materials 710 (Sept. 2016), pp. 244–249.
- [86] J. M. Sanchez, M. Arribas, H. Galarraga, M. Garcia de Cortazar, M. Ellero, and F. Girot. “Efects of Mn addittion, cooling rate and holding temperature on the modifcation and purifcation of iron-rich compounds in AlSi10MnMg(Fe) alloy”. In: Heliyon 9.2 (Feb. 2023), e13005.
- [87] E. Cinkilic, C. D. Ridgeway, X. Yan, and A. A. Luo. “A Formation Map of IronContaining Intermetallic Phases in Recycled Cast Aluminum Alloys”. In: Metallurgical and Materials Transactions A 50.12 (Dec. 2019), pp. 5945–5956.
- [88] J. Piatkowski, M. Hejne, and R. Wiesza la. “Infuence of manganese content on the microstructure and properties of AlSi10MnMg(Fe) alloy for die castings”. In: Archives of Materials Science and Engineering 123.1 (Sept. 2023), pp. 5–12.
- [89] A. Niklas, A. Bakedano, S. Orden, M. da Silva, E. Nogu´es, and A. Fern´andezCalvo. “Efect of Microstructure and Casting Defects on the Mechanical Properties of Secondary AlSi10MnMg(Fe) Test Parts Manufactured by Vacuum Assisted high Pressure Die Casting Technology”. In: Materials Today: Proceedings 2.10 (2015), pp. 4931–4938.
- [90] B. Dalai, S. Jonsson, M. da Silva, P. ˚Akerstr¨om, and J. Kajberg. “Microstructure Evolution, Mechanical Properties and Fracture Analysis of a High-Pressure DieCast Secondary AlSi10MnMg(Fe) Alloy”. In: 9th International Conference on Hot Sheet Metal Forming of High-Performance Steel. May. AIST, 2024, pp. 319–324.
- [91] X. Jiao, P. Wang, Y. Liu, J. Wang, W. Liu, A. Wan, L. Shi, C. Wang, and S. Xiong. “Fracture behavior of a high pressure die casting AlSi10MnMg alloy with varied porosity levels”. In: Journal of Materials Research and Technology 25 (July 2023), pp. 1129–1140.
- [92] A. K. M. A. Ahamed and H. Kato. “Infuence of Casting Defects on Tensile Properties of ADC12 Aluminum Alloy Die-Castings”. In: MATERIALS TRANSACTIONS 49.7 (2008), pp. 1621–1628.
- [93] B. Dybowski, A. Kie lbus, and L. Poloczek. “Efects of die-casting defects on the blister formation in high-pressure die-casting aluminum structural components”. In: Engineering Failure Analysis 150.February (Aug. 2023), p. 107223.
- [94] H. Yang, Z. Guo, and S. Xiong. “Microstructure and mechanical properties of highpressure die cast pure copper”. In: Journal of Materials Processing Technology 275.August 2019 (Jan. 2020), p. 116377.
- [95] Z. Yuan, Z. Guo, and S. Xiong. “Skin layer of A380 aluminium alloy die castings and its blistering during solution treatment”. In: Journal of Materials Science & Technology 35.9 (Sept. 2019), pp. 1906–1916.
- [96] S. Otarawanna, C. Gourlay, H. Laukli, and A. Dahle. “Formation of the surface layer in hypoeutectic Al-alloy high-pressure die castings”. In: Materials Chemistry and Physics 130.1-2 (Oct. 2011), pp. 251–258.
- [97] H. Zheng, Y. Jiang, F. Liu, and H. Zhao. “Microstructure heterogeneity optimization of HPDC Al-Si-Mg-Cu alloys by modifying the characteristic of externally solidifed crystals”. In: Journal of Alloys and Compounds 976.November 2023 (Mar. 2024), p. 173167.
- [98] B. Dalai. Material characterization of AA7075-T651 deformed at diferent temperatures and strain rates. Tech. rep. Lule˚a: Lule˚a University of Technology, 2021.
- [99] B. Hopkinson. “X. A method of measuring the pressure produced in the detonation of high, explosives or by the impact of bullets”. In: Philosophical Transactions of the Royal Society of London. Series A, Containing Papers of a Mathematical or Physical Character 213.497-508 (Jan. 1914), pp. 437–456.
- [100] R. M. Davies. “A critical study of the Hopkinson pressure bar”. In: Philosophical Transactions of the Royal Society of London. Series A, Mathematical and Physical Sciences 240.821 (Jan. 1948), pp. 375–457.
- [101] H. Kolsky. “An Investigation of the Mechanical Properties of Materials at very High Rates of Loading”. In: Proceedings of the Physical Society. Section B 62.11 (Nov. 1949), pp. 676–700.
- [102] J. Kajberg and K. G. Sundin. “Material characterisation using high-temperature Split Hopkinson pressure bar”. In: Journal of Materials Processing Technology 213.4 (2013), pp. 522–531.
- [103] J. Kajberg and K. Sundin. “High-Temperature Split-Hopkinson Pressure Bar with a Momentum Trap for Obtaining Flow Stress Behaviour and Dynamic Recrystallisation”. In: Strain 50.6 (Dec. 2014), pp. 547–554.
- [104] V. Pare, S. Modi, and K. Jonnalagadda. “Thermo-mechanical behavior and bulk texture studies on AA5052-H32 under dynamic compression”. In: Materials Science and Engineering: A 668 (June 2016), pp. 38–49.
- [105] W.-S. Lee and C.-F. Lin. “Plastic deformation and fracture behaviour of Ti–6Al–4V alloy loaded with high strain rate under various temperatures”. In: Materials Science and Engineering: A 241.1-2 (Jan. 1998), pp. 48–59.
9. 著作権:
*この資料は、M. WessénおよびL. Näslundの論文:「Influence of Die Temperature in High Pressure Die Casting of Thin-Walled Components」に基づいています。
*論文ソース: https://ltu.diva-portal.org/smash/get/diva2:1901057/FULLTEXT01.pdf
この資料は上記の論文に基づいて要約されたものであり、商業目的での無断使用は禁止されています。
Copyright © 2025 CASTMAN. All rights reserved.