この論文の要約は、"Improving Electrical Conductivity of Commercially Pure Aluminium: The Synergistic Effect of AlB8 Master Alloy and Heat Treatment"と題された論文に基づいており、"Materials (MDPI)"で発表されました。
1. 概要:
- タイトル: 市販純アルミニウムの電気伝導率の向上:AlB8マスター合金と熱処理の相乗効果
- 著者: ユスフ・ゼイベック、セミーレ・カユシュ、エゲ・アヌル・ディレル
- 発表年: 2025年
- 掲載ジャーナル: Materials (MDPI)
- キーワード: 市販純アルミニウム、電気伝導率、アルミニウム-ホウ素マスター合金、結晶粒粗大化熱処理、電気モーター効率
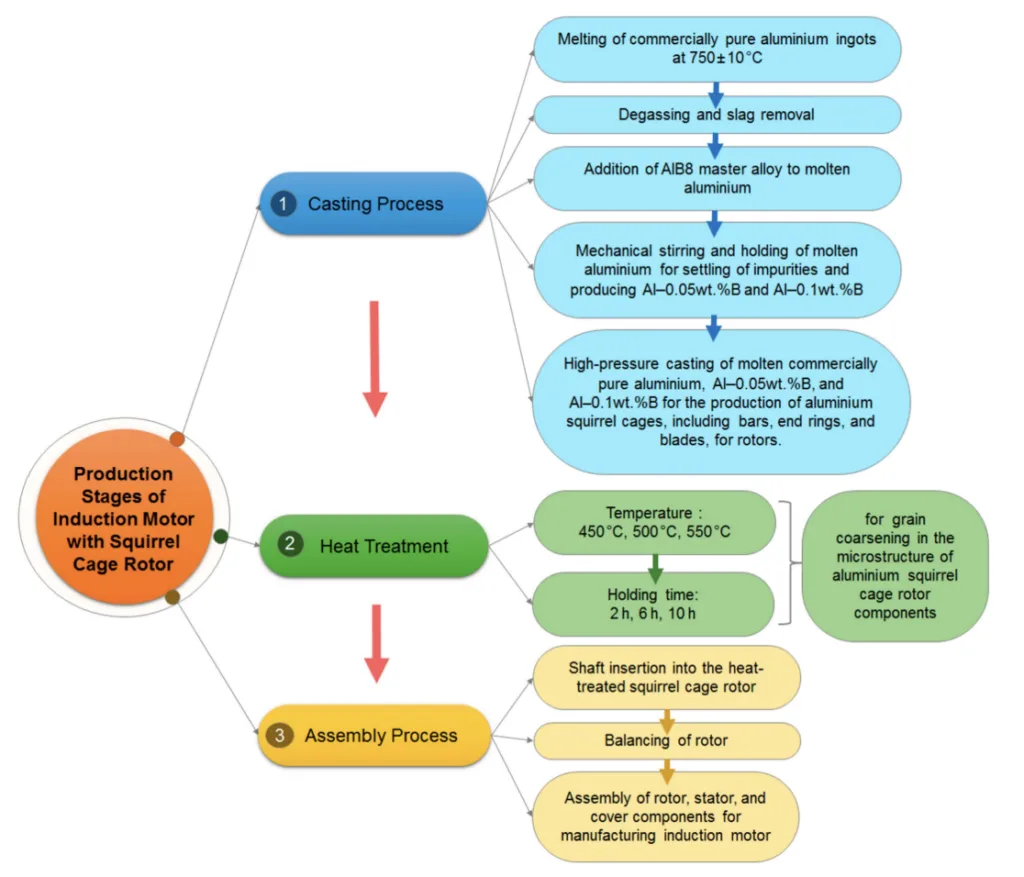
2. 研究背景:
世界的なエネルギー需要の増大と温室効果ガス排出量削減の必要性が高まる中で、エネルギー効率の向上が最重要課題となっています。電気モーターは、産業および商業分野で広く利用されており、効率改善の大きな機会を提供しています。市販純アルミニウム(CP-Al)は、低密度とコスト効率の高さから、誘導モーターのリスケージローターの製造によく使用されています。しかし、アルミニウム固有の電気伝導率は高いものの、モーター性能を向上させるためにさらに最適化することが可能です。
アルミニウムの電気伝導率を向上させる既存の方法としては、希土類元素の利用が挙げられます。これらの方法は効果的ですが、コストが大幅にかかります。アルミニウム-ホウ素(Al-B)マスター合金は、アルミニウム中の不純物低減のためのより経済的な代替手段を提供します。しかし、Al-Bマスター合金の適用は結晶粒微細化を引き起こす可能性があり、結晶粒界散乱の増加により、電気伝導率の望ましい向上を相殺する可能性があります。したがって、Al-Bマスター合金の不純物除去能力を活用するだけでなく、結晶粒微細化効果を軽減し、電気モーター用途向けのCP-Alの電気伝導率を最大化する費用対効果の高い戦略が不可欠です。
3. 研究目的と研究課題:
本研究は、市販純アルミニウムの電気伝導率を大幅に向上させることを目的としています。主な目的は、アルミニウムの微細構造内の不純物と結晶粒界の両方を最小限に抑え、この材料で作られたリスケージローターを利用する電気モーターの効率を向上させることです。
本研究で取り組む主な研究課題は以下のとおりです。
- AlB8マスター合金の添加は、市販純アルミニウムの純度レベルと電気伝導率にどのような影響を与えるか?
- 結晶粒粗大化熱処理は、市販純アルミニウムの結晶粒径と電気伝導率にどのような影響を与えるか?
- 市販純アルミニウムの電気伝導率を向上させる上で、AlB8マスター合金の添加と結晶粒粗大化熱処理の間に相乗効果は存在するか?
- 電気伝導率の向上は、電気モーターの効率向上にどの程度つながるか?
中心となる研究仮説は、AlB8マスター合金の添加とそれに続く結晶粒粗大化熱処理の組み合わせ適用が、市販純アルミニウムの電気伝導率を相乗的に大幅に向上させ、最終的に電気モーターの効率の測定可能な向上につながるであろうというものです。
4. 研究方法
本研究では、統計的実験計画法と応答曲面法に基づいた厳密な研究方法を採用し、Box–Behnken計画を利用しました。
- 研究デザイン: ホウ素添加量、熱処理温度、保持時間の3つの因子が、市販純アルミニウムの電気伝導率に及ぼす影響を調査するために、Box–Behnken実験計画法を実施しました。
- データ収集方法: 電気伝導率の測定は、DIN EN 2004–1およびASTM E1004-17規格に従い、渦電流法を用いたSIGMASCOPE® SMP10装置を使用して実施しました。微細構造特性評価は光学顕微鏡を用いて行い、結晶粒径解析はImageJソフトウェアを用いて実施しました。モーター性能評価は、KISTLERモーター試験装置を用いて実施しました。化学組成分析は、発光分光分析法を用いて行いました。
- 分析方法: Design Expert Version 13ソフトウェアによって促進された分散分析(ANOVA)および回帰分析を利用して、電気伝導率に対する因子とその相互作用の統計的有意性を評価しました。変数と応答の間の関係を視覚化するために、応答曲面プロットと等高線プロットを作成しました。
- 研究対象と範囲: 研究対象は、純度99.7%の市販純アルミニウムに焦点を当てました。AlB8マスター合金を導入して、ホウ素添加量を0.05 wt.%および0.1 wt.%にしました。リスケージローターは、高圧ダイカスト法を用いて製造しました。結晶粒粗大化熱処理は、450 °C、500 °C、および550 °Cの温度で、2時間、6時間、および10時間の保持時間で実施しました。
5. 主な研究結果:
実験結果は、AlB8マスター合金の添加と結晶粒粗大化熱処理の相乗的な適用により、市販純アルミニウムの電気伝導率が大幅に向上することを示しました。
- 主な研究結果: 電気伝導率の最も顕著な向上は、0.05 wt.%のホウ素添加と550 °Cで10時間の結晶粒粗大化熱処理の組み合わせで達成されました。このハイブリッドアプローチにより、電気伝導率は初期の60.62% IACSから63.1% IACSに向上しました。対応して、この方法で製造されたローターを使用して製造された電気モーターの効率は、90.35%から91.53%に向上しました。スペクトル分析により、ホウ素添加が不純物、特にチタン(Ti)、バナジウム(V)、ジルコニウム(Zr)などの遷移金属を効果的に低減することが確認されました。微細構造解析により、熱処理が結晶粒粗大化を効果的に促進し、結晶粒界密度を低減することが明らかになりました。
- 統計的/定性的分析結果: ANOVA分析の結果、ホウ素添加、熱処理温度、保持時間、およびそれらの二次効果と相互作用効果(ホウ素添加と保持時間の間の相互作用を除く)が、電気伝導率に有意な影響を与えていることが示されました(p < 0.05)。回帰モデルは、高い決定係数(R² = 0.9859)を示し、モデルの適合性が高いことを示しています。
- データ解釈: 電気伝導率の向上は、ホウ素添加による不純物除去と、結晶粒粗大化熱処理による結晶粒界散乱の低減の複合効果に起因すると考えられます。相乗効果は、優れた電気性能を達成するためには、材料の純度と微細構造の両方を最適化することが重要であることを強調しています。
図表リスト:
- 図1. リスケージローターの製造工程、熱処理の適用、およびその後の誘導電動機の製造工程を示す図。
- 図2. ホウ素添加による不純物除去の模式図。
- 図3. 高圧ダイカスト法により市販純アルミニウムから製造されたリスケージローターとその構成部品。
- 図4. リスケージローターを備えた誘導電動機の組立図。
- 図5. アルミニウム試料の電気伝導率測定。
- 図6. 誘導電動機の性能試験。
- 図7. 中心点(0,0,0)と3つの因子の12個の要因点を持つBox-Behnken計画キューブ。
- 図8. 未処理および熱処理されたアルミニウム試料の光学顕微鏡写真。
- 図9. 未処理アルミニウム試料の結晶粒径解析。
- 図10. 熱処理されたAl-0.05B試料の結晶粒径解析。
- 図11. 電気伝導率に影響を与える線形、二次、および相互作用項の寄与率。
- 図12. 電気伝導率の予測値と実験値の相関グラフ。
- 図13. 電気伝導率に対するホウ素添加、熱処理温度、および保持時間の主効果プロット。
- 図14. 電気伝導率に対する相互作用の効果を示す2次元等高線プロットと3次元応答曲面プロット。
- 図15. 未処理および熱処理されたCP-Al、Al-0.05B、およびAl-0.1Bのモーター効率と電気伝導率の関係。
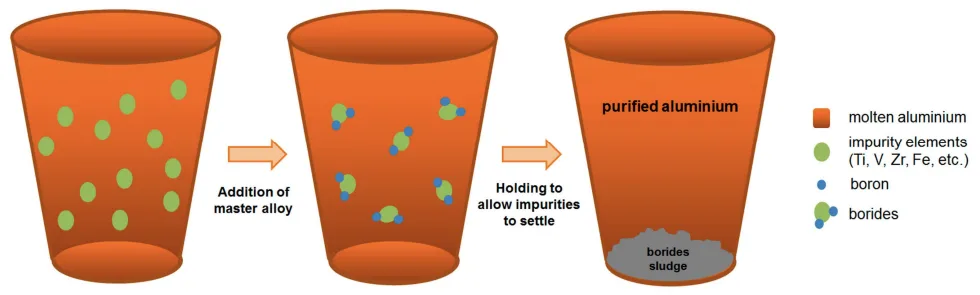
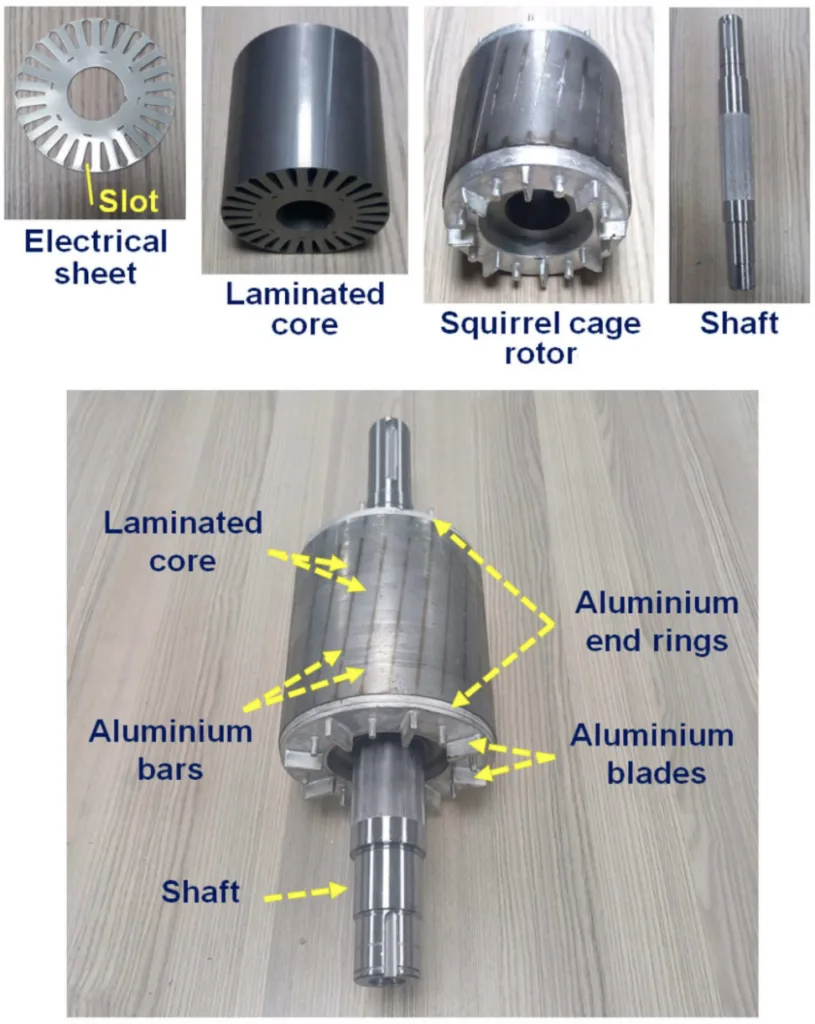
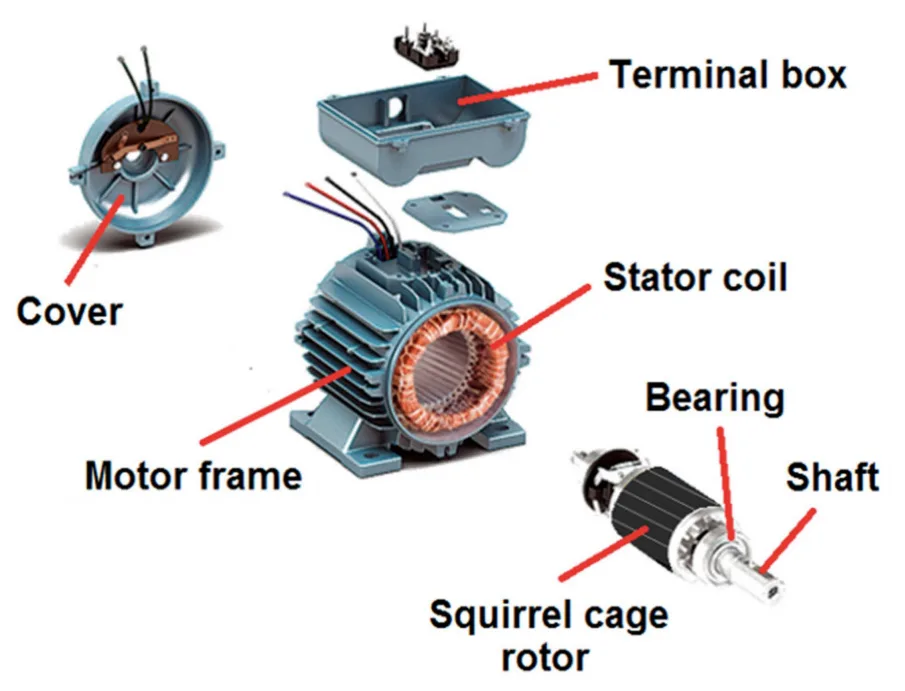
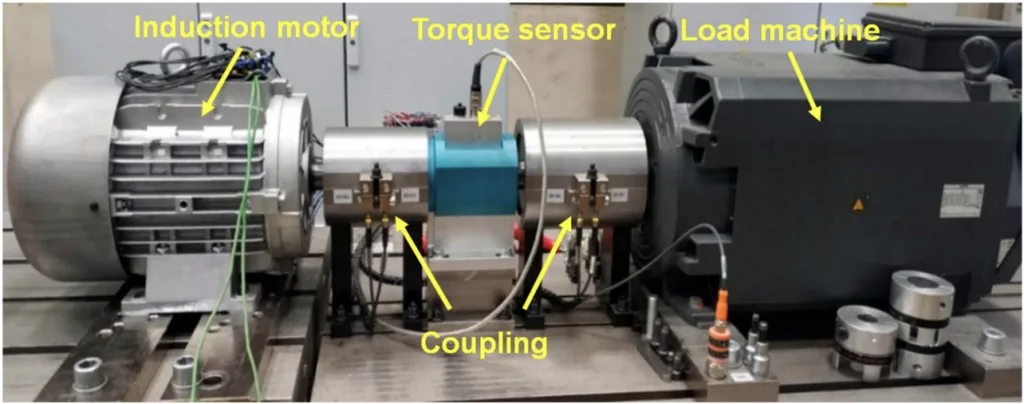
6. 結論と考察:
- 主な結果の要約: 本研究では、AlB8マスター合金の添加と結晶粒粗大化熱処理を組み合わせたハイブリッドアプローチが、市販純アルミニウムの電気伝導率を向上させる効果的な方法であり、電気モーターの効率を著しく向上させることを実証しました。
- 研究の学術的意義: 本研究は、金属の伝導率を最適化するための相乗的な材料加工技術の基礎的理解に貢献します。強化された電気特性を達成する上での不純物制御と微細構造工学の相互作用を強調しています。
- 実用的な意義: 本研究の知見は、市販純アルミニウムを利用した電気モーターの性能を向上させるための、実行可能で費用対効果の高い方法を提供します。このアプローチは、さまざまな産業用途におけるエネルギー効率の向上と、持続可能な製造慣行の促進に大きな実用的な意義を持ちます。
- 研究の限界: 本研究は電気伝導率の向上に効果的に取り組みましたが、改質されたアルミニウムの機械的特性には主に焦点を当てていません。高伝導率であっても、純アルミニウムの適用性は、高い機械的強度を必要とするシナリオでは制限される可能性があります。
7. 今後のフォローアップ研究:
- 今後のフォローアップ研究の方向性: 今後の研究では、達成された電気伝導率を維持または向上させながら、市販純アルミニウムの機械的特性をさらに向上させることに焦点を当てる必要があります。これには、異なる合金元素の添加や、代替の熱処理条件の検討が含まれる可能性があります。
- さらなる探求が必要な分野: 熱伝導率や耐食性など、他の重要な材料特性に対するこのハイブリッドアプローチの影響を評価するために、さらなる調査が必要です。自動車やエネルギーなど、多様な分野におけるこの強化された市販純アルミニウムのより広範な産業用途を探求することも推奨されます。
8. 参考文献:
- [1] De Almeida, A.T.; Ferreira, F.J.T.E.; Fong, J. Perspectives on electric motor market transformation for a net zero carbon economy. Energies 2023, 16, 1248.
- [2] Li, Z.; Hamidi, A.S.; Yan, Z.; Sattar, A.; Hazra, S.; Soulard, J.; Guest, C.; Ahmed, S.H.; Tailor, F. A circular economy approach for recycling electric motors in the end-of-life vehicles: A literature review. Resour. Conserv. Recycl. 2024, 205, 107582.
- [3] Mahale, M.D.; Patra, B.B. Efficient design, materials and specifications of electric motors used in electric vehicle challenges. In Energy Efficient Vehicles: Technologies and Challenges, 1st ed.; Singh, V.P., Kumar, A., Meena, C.S., Dwivedi, G., Eds.; CRC Press: Boca Raton, FL, USA, 2024; pp. 126–155.
- [4] Paul, K.; Sharma, D.; Konda, K.R.; Xie, T.; Höschler, K. Comprehensive review on cooling of permanent magnet synchronous motors and their qualitative assessment for aerospace applications. Energies 2023, 16, 7524.
- [5] Grassmann, R.M.; Shentu, C.; Hamoda, T.; Dewi, P.T.; Burgner-Kahrs, J. Open continuum robotics-one actuation module to create them all. Front. Robot. Al 2024, 11, 1272403.
- [6] Sun, B.; Zhang, Q.; Mao, H.; Li, Z. Validation of a statistical-dynamic framework for predicting energy consumption: A study on vehicle energy conservation equation. Energy Convers. Manag. 2024, 307, 118330.
- [7] Krzysztof, P.; Wnęk, H. Analysis of energy flow in hybrid and electric-drive vehicles. Energies 2024, 17, 1915.
- [8] Krishnamoorthy, S.; Panikkar, P.P.K. A comprehensive review of different electric motors for electric vehicles application. Int. J. Power Electron. Drive Syst. 2024, 15, 74–90.
- [9] Tong, W. Mechanical Design and Manufacturing of Electric Motors, 2nd ed.; CRC Press: Boca Raton, FL, USA, 2022; pp. 1–94.
- [10] Gobbi, M.; Sattar, A.; Palazzetti, R.; Mastinu, G. Traction motors for electric vehicles: Maximization of mechanical efficiency—A review. Appl. Energy 2024, 357, 122496.
- [11] Kumar, P.; Channi, H.K.; Singh, H. A Comprehensive Review of Electrical Motors for Electric Mobility. In Proceedings of 2024 IEEE International Conference for Women in Innovation, Technology & Entrepreneurship (ICWITE), Bangalore, India, 16–17 February 2024; pp. 130–135.
- [12] Manohar, V.J. Advantages and disadvantages of brushless DC motor. In Electrical Devices & Systems; Reddy, K.S., Ed.; CIIR Research Publications: Uttar Pradesh, India, 2023; pp. 20–22.
- [13] Mishra, A.; Agarwal, P.; Srivastava, S.P. A comprehensive analysis and implementation of vector control of permanent magnet synchronous motor. Int. J. Power Energy Convers. 2014, 5, 1–23.
- [14] Pindoriya, R.M.; Rajpurohit, B.S.; Kumar, R.; Srivastava, K.N. Comparative Analysis of Permanent Magnet Motors and Switched Reluctance Motors Capabilities for Electric and Hybrid Electric Vehicles. In Proceedings of 2018 IEEMA Engineer Infinite Conference (eTechNxT), New Delhi, India, 13–14 March 2018.
- [15] Jape, S.R.; Thosar, A. Comparison of electric motors for electric vehicle application. Int. J. Res. Eng. Technol. 2017, 6, 12–17.
- [16] Dimitrios, R.; Kaminaris, S.D.; Piromalis, D.D.; Vokas, G.; Arvanitis, K.G.; Karavas, C.S. Comparative review of motor technologies for electric vehicles powered by a hybrid energy storage system based on multi-criteria analysis. Energies 2023, 16, 2555.
- [17] Basma, B.; Cheghib, H.; Chrenko, D.; Delgado, M.T.; Hamoudi, Y.; Rodriguez, J.; Abdelrahem, M. Sliding mode control of an electric vehicle driven by a new powertrain technology based on a dual-star induction machine. World Electr. Veh. J. 2024, 15, 155.
- [18] Kurt, M.Ş. The use of induction motors in electric vehicles. In Induction Motors—Recent Advances, New Perspectives and Applications; El-Shahat, A., Ed.; IntechOpen: London, UK, 2023; pp. 1–10.
- [19] Thirugnanam, P. Advances, new perspective and applications in induction motors. In Induction Motors—Recent Advances, New Perspectives and Applications; El-Shahat, A., Ed.; IntechOpen: London, UK, 2023; pp. 1–15.
- [20] Su, H.; Wen, H.; Zheng, X.; Su, J. Development of a super high efficiency motor with boron aluminum alloy rotor. Procedia Eng. 2017, 174, 1221–1228.
- [21] Shadfari, H.; Izadfar, H.R. A new squirrel cage rotor structure to improve the dynamic performance of the single-phase induction motor. Int. J. Ind. Electron. Control. Optim. 2022, 5, 279–286.
- [22] Nandagopal, S.; Chokkalingam, L.N. Influence of squirrel cage induction rotor geometry in battery C-rating. Eng. Sci. Technol. 2023, 39, 101336.
- [23] Luthfiyah, H.; Qowiy, O.A.; Malakani, A.I.; Arthanto, D.H.; Setiawan, F.D.; Ramanel, T.A.; Putra, G.M.; Kamar, S.; Suryandi, A.A. An optimized stator and rotor design of squirrel cage induction motor for EMU train. J. Mechatron. Electr. Power Veh. Technol. 2023, 14, 35–46.
- [24] Wang, L.; Bao, X.; Di, C.; Zhou, Y. Influence on vibration and noise of squirrel-cage induction machine with double skewed rotor for different slot combinations. IEEE Trans. Magn. 2016, 52, 8104404.
- [25] Balcı, M.Ş.; Dalcalı, A. The effect of rotor slot number on the performance of asynchronous motors in light electric vehicle. Aintelia Sci. Notes 2023, 2, 40–46.
- [26] Dinh, B.M. Efficiency Improvement of Squirrel Cage Induction Motor by Rotor Slot Designs. In Proceedings of 13th International Conference on Industrial Engineering and Operations Management, Manila, Philippines, 7–9 March 2023; IEOM Society International: Manila, Philippines, 2023; pp. 615–623.
- [27] Konda, R.Y.; Ponnaganti, V.K.; Reddy, P.V.S.; Singh, R.R.; Mercorelli, P.; Gundabattini, E.; Solomon, D.G. Thermal analysis and cooling strategies of high-efficiency three-phase squirrel-cage induction motors—A review. Computation 2024, 12, 6.
- [28] Özsoy, M.; Kaplan, O.; Akar, M. FEM-Based Analysis of rotor cage material and slot geometry on double air gap axial flux induction motors. Ain Shams Eng. J. 2024, 15, 102393.
- [29] Marfoli, A.; Nardo, M.D.; Degano, M.; Gerada, C.; Jara, W. Squirrel cage induction motor: A design-based comparison between aluminium and copper cages. IEEE Open J. Ind. Appl. 2021, 2, 110–120.
- [30] Liu, Y.; Han, P.; Bazzi, A.M. A Comparison of Rotor Bar Material of Squirrel-Cage Induction Machines for Efficiency Enhancement Purposes. In Proceedings of 2015 17th European Conference on Power Electronics and Applications (EPE’15 ECCE-Europe), Geneva, Switzerland, 8–10 September 2015.
- [31] Ocak, C. A FEM-based comparative study of the effect of rotor bar designs on the performance of squirrel cage induction motors. Energies 2023, 16, 6047.
- [32] Agapiou, J.S. Development of manufacturing technology for a hybrid induction rotor. Manuf. Lett. 2023, 35, 277–288.
- [33] Mallard, V.; Parent, G.; Demian, C.; Brudny, J.-F.; Delamotte, A. Increasing the energy efficiency of induction machines by the use of grain-oriented magnetic materials and die casting copper squirrel cage in the rotor. IEEE Trans. Ind. Appl. 2019, 55, 1280–1289.
- [34] Peters, D.T.; Cowie, J.G.; Brush, E.F. Die casting copper motor rotors: Mold materials and processing for cost-effective manufacturing. In Energy Efficiency Improvements in Electronic Motors and Drives; Bertoldi, P., De Almeida, A.T., Falkner, H., Eds.; Springer: Berlin, Germany, 2000; pp. 39–51.
- [35] Peters, D.T.; Cowie, J.G.; Brush, E.F.; Midson, S.P. Die-cast copper motor rotors: Die materials and process considerations for economical copper rotor production. In Energy Efficiency in Motor Driven Systems; Parasiliti, F., Bertoldi, P., Eds.; Springer: Berlin, Germany, 2003; pp. 128–135.
- [36] Feng, Y.N.; Apsley, J.; Williamson, S.; Smith, A.C.; Ionel, D.M. Reduced losses in die-cast machines with insulated rotors. IEEE Trans. Ind. Appl. 2010, 46, 928–936.
- [37] Varghese, S.T.; Singh, B.; Rajagopal, K.R. Fault investigations on die-cast copper rotors. IEEE Trans. Ind. Appl. 2018, 54, 184–194.
- [38] Agapiou, J.S. Development on die-cast copper motor rotors—Casting considerations. Manuf. Lett. 2022, 33, 310–321.
- [39] Cowie, J.G.; Brender, D.T. Die-Cast Copper Rotors for Improved Motor Performance. In Proceedings of the Conference Record of the 2003 Annual Pulp and Paper Industry Technical Conference, Charleston, SC, USA, 16–20 June 2003; pp. 42–49.
- [40] Passarini, F.; Ciacci, L.; Nuss, P.; Manfredi, S. Material Flow Analysis of Aluminium, Copper, and Iron in the EU-28. EUR 29220 EN; Publications Office of the European Union: Luxembourg, 2018; ISBN 978-92-79-85744-7.
- [41] Lin, G.; Li, L.; Guo, Z.; Jia, X.; Wang, X.; Yuan, Z.; Zhang, G.; Zhan, Y.; Shan, Q.; Li, Z. Influence of cerium and yttrium addition on strength and electrical conductivity of pure aluminum alloys. J. Rare Earth. 2024, 42, 600–611.
- [42] Zhang, Y.; Wei, F.; Mao, J.; Niu, G. The difference of La and Ce as additives of electrical conductivity aluminum alloys. Mater. Charact. 2019, 158, 109963.
- [43] Medvedev, A.E.; Murashkin, M.Y.; Enikeev, N.A.; Valiev, R.Z.; Hodgson, P.D.; Lapovok, R. Enhancement of mechanical and electrical properties of Al-RE alloys by optimizing rare-earth concentration and thermo-mechanical treatment. J. Alloys Compd. 2018, 745, 696–704.
- [44] Murashkin, M.Y.; Sabirov, I.; Medvedev, A.E.; Enikeev, N.A.; Lefebvre, W.; Valiev, R.Z.; Sauvage, X. Mechanical and electrical properties of an ultrafine grained Al–8.5wt. % RE (RE=5.4wt.% Ce, 3.1wt.% La) alloy processed by severe plastic deformation. Mater. Des. 2016, 90, 433–442.
- [45] Cui, X.; Wu, Y.; Liu, X.; Zhao, Q.; Zhang, G. Effects of grain refinement and boron treatment on electrical conductivity and mechanical properties of AA1070 aluminum. Mater. Des. 2015, 86, 397–403.
- [46] Cui, X.; Wu, Y.; Cui, H.; Zhang, G.; Zhou, B.; Liu, X. The improvement of boron treatment efficiency and electrical conductivity of AA1070Al achieved by trace Ti assistant. J. Alloys Compd. 2018, 735, 62–67.
- [47] Karabay, S.; Uzman, I. Inoculation of transition elements by addition of AlB2 and AlB12 to decrease detrimental effect on the conductivity of 99.6% aluminium in CCL for manufacturing of conductor. J. Mater. Process. Technol. 2005, 160, 174–182.
- [48] Xu, X.; Feng, Y.; Fan, H.; Wang, Q.; Dong, G.; Li, G.; Zhang, Z.; Liu, Q.; Fan, X.; Ding, H. The grain refinement of 1070 alloy by different Al-Ti-B mater alloys and its influence on the electrical conductivity. Results Phys. 2019, 14, 102482.
- [49] Wang, T.; Chen, Z.; Fu, H.; Gao, L.; Li, T. Grain refinement mechanism of pure aluminum by inoculation with Al-B master alloys. Mater. Sci. Eng. A 2012, 549, 136–143.
- [50] Wang, T.; Chen, Z.; Fu, H.; Xu, J.; Fu, Y.; Li, T. Grain refining potency of Al-B master alloy on pure aluminum. Scr. Mater. 2011, 64, 1121–1124.
- [51] Dong, L.; Yang, F.; Yu, T.; Zhang, N.; Zhou, X.; Xie, Z.; Fang, F. Contribution of grain boundary to strength and electrical conductivity of annealed copper wires. J. Mater. Res. Technol. 2023, 26, 1459–1468.
- [52] Bakonyi, I. Accounting for the resistivity contribution of grain boundaries in metals: Critical analysis of reported experimental and theoretical data for Ni and Cu. Eur. Phys. J. Plus 2021, 136, 410.
- [53] Zheng, Q.; Zhang, B.; Chen, T.; Wu, J. Achieving superior grain refinement efficiency for Al-Si casting alloys through a novel Al-La-B grain refiner. J. Mater. Res. Technol. 2024, 30, 52–60.
- [54] Liu, G.; Ren, Y.; Ma, W.; Morita, K.; Lei, Y.; Zhan, S.; Lv, G.; Li, S.; Zeng, Y.; Li, R. Development process and future trends of chemical refining agents' influence on grain refinement in aluminum alloys. J. Mater. Res. Technol. 2024, 29, 242–257.
- [55] Sigworth, G.K. Grain refinement of Al-Si-Cu alloys by AlB2 and (Al,Ti)B2. Inter. Metalcast. 2024, 18, 2778–2782.
- [56] Birol, Y. Grain Refining Aluminium foundry alloys with commercial Al-B master alloys. Mater. Sci. Technol. 2014, 30, 277–282.
- [57] Khaliq, A.; Rhamdhani, M.A.; Brooks, G.A.; Grandfield, J. Analysis of boron treatment for V removal using AlB2 and AlB12 based master alloys. In Light Metals 2014; Grandfield, J., Ed.; Springer: Cham, Switzerland, 2014; pp. 963–968.
- [58] Yabiku, R.; Fialho, R.; Teran, L.; Santos, A.; Rangel, E.; Dutra, D. A Comparative Study between Copper and Aluminum Induction Squirrel Cage Constructions. In 2010 Record of Conference Papers Industry Applications Society 57th Annual Petroleum and Chemical Industry Conference (PCIC), San Antonio, TX, USA, 20–22 September 2010; pp. 1–9.
- [59] Czerwinski, F. Aluminum alloys for electrical engineering: A review. J. Mater. Sci. 2024, 59, 14847–14892.
- [60] Aboraia, M.; Elrab, H.G.G.; Abdalla, G. Joint addition of zirconium, titanium and chromium to commercial pure aluminium. J. P. Min. Eng. 2020, 22, 58–64.
- [61] Liu, J.; Wang, Y.; Cui, X. Study about the forming mechanism of boron in Al-1B alloy. Met. Mater. Int. 2024, in press.
- [62] Khaliq, A.; Rhamdhani, M.A.; Mitchell, J.B.; Davidson, C.J. Analysis of Transition Metal (V, Zr) Borides Formation in Aluminium Melt. In Proceedings of European Metallurgical Conference (EMC) 2011, Dusseldorf, Germany, 26–29 June 2011.
- [63] Khaliq, A.; Rhamdhani, M.A.; Brooks, G.A.; Grandfield, J. Performance Evaluation of AlB12 and AlB2 for the Boron Treatment of Molten Aluminium. In Proceedings of the 6th Annual High Temperature Processing Symposium 2014, Melbourne, Australia, 3–4 February 2014; pp. 75–78.
- [64] Barta, J.; Uzhegov, N.; Losak, P.; Ondrusek, C.; Mach, M.; Pyrhönen, J. Squirrel-cage rotor design and manufacturing for high-speed applications. IEEE Trans. Ind. Electron. 2019, 66, 6768–6778.
- [65] Gerada, D.; Mebarki, A.; Brown, N.L.; Bradley, K.J.; Gerada, C. Design aspects of high-speed high-power-density laminated-rotor induction machines. IEEE Trans. Ind. Electron. 2011, 58, 4039–4047.
- [66] Jo, S.H.; Lee, S.H. Changes in microstructure, mechanical properties, and electrical conductivity of severely drawn AA1070 wire with annealing. Korean J. Met. Mater. 2024, 62, 749–756.
- [67] Wang, Q.; Yang, P.; Zhang, B.; Fan, H.; Xu, X.; Li, W.; Fan, X.; Wang, J.; Ding, H. Microstructure and texture evolution of cold rolled 1070 Al alloy during the subsequent annealing treatment. Results Phys. 2019, 13, 102178.
- [68] DIN EN 2004-1; Aerospace Series; Test Methods for Aluminium and Aluminium Alloy Products; Part 1: Determination of Electrical Conductivity of Wrought Aluminium Alloys; German Institute for Standardisation: Berlin, Germany, 1993.
- [69] ASTM E1004-17; Test Method for Determining Electrical Conductivity Using the Electromagnetic (Eddy-Current) Method; ASTM International: West Conshohocken, PA, USA, 2017.
- [70] IACS. International Annealed Copper Standard (IACS); United States Department of Commerce: Washington, DC, USA, 1914.
- [71] TS EN 60034-2-1; Rotating Electrical Machines - Part 2-1: Standard Methods for Determining Losses and Efficiency from Tests (Excluding Machines for Traction Vehicles); Turkish Standards Institution: Ankara, Turkey, 2014.
- [72] ISO/IEC 17025:2017; General Requirements for the Competence of Testing and Calibration Laboratories; International Organization for Standardization and International Electrotechnical Commission: Geneva, Switzerland, 2017.
- [73] Olabinjo, O.O. Response surface techniques as an inevitable tool in optimization process. In Response Surface Methods—Theory, Applications and Optimization Techniques; Silva, V., Cardoso, J.S., Eds.; IntechOpen: London, UK, 2024; pp. 1–11.
- [74] Ferreira, S.L.C.; Bruns, R.E.; Ferreira, H.S.; Matos, G.D.; David, J.M.; Brandão, G.C.; da Silva, E.G.P.; Portugal, L.A.; dos Reis, P.S.; Souza, A.S.; et al. Box-Behnken design: An alternative for the optimization of analytical methods. Anal. Chim. Acta 2007, 597, 179–186.
- [75] Myers, R.H.; Montgomery, D.C.; Anderson-Cook, C.M. Response Surface Methodology: Process and Product Optimization Using Designed Experiments, 4th ed.; John Wiley & Sons: Hoboken, NJ, USA, 2016; pp. 1–825.
- [76] Iwundu, M.P.; Cosmos, J. The efficiency of seven-variable Box-Behnken experimental design with varying center runs on full and reduced model types. J. Math. Stat. 2022, 18, 196–207.
- [77] Elkady, E.F.; Fouad, M.A.; Mozayad, A.N. Application of Box-Behnken experimental design and response surface methodology for selecting the optimum RP-HPLC conditions for the simultaneous determination of methocarbamol, indomethacin and betamethasone in their pharmaceutical dosage form. BMC Chem. 2022, 6, 114.
- [78] Draper, N.R. Center points in second-order response surface designs. Technometrics 1982, 24, 127–133.
- [79] Hemavathi, M.; Varghese, E.; Shekhar, S.; Jaggi, S.; Bhowmik, A.; Sathianandan, T.V. Run order consideration for sequential third order rotatable designs. Commun. Stat. Simul. Comput. 2024, 53, 1068–1081.
- [80] Cooper, P.S.; Kearns, M.A. Removal of transition metal impurities in aluminium melts by boron additives. Mater. Sci. Forum 1996, 217-222, 141–146.
- [81] Khaliq, A. Thermodynamics and Kinetics of Transition Metal Borides Formation in Molten Aluminium. Ph.D. Thesis, Swinburne University of Technology, Melbourne, Australia, 2013.
- [82] Khaliq, A.; Rhamdhani, M.A.; Brooks, G.A.; Grandfield, J. Thermodynamic analysis of Ti, Zr, V and Cr impurities in aluminum melt. In Light Metals 2011; Lindsay, S.J., Ed.; Springer: Cham, Switzerland, 2011; pp. 751–756.
- [83] Gao, J.W.; Shu, D.; Wang, J.; Sun, B.D. Effect of boron on removal of iron from aluminium melts. Mater. Sci. Technol. 2009, 25, 1462–1466.
- [84] Imam, M.A. Phase Equilibria and Reaction Kinetics of Borides Based High-Temperature Thermoelectric Materials. Ph.D. Thesis, The University of Alabama, Tuscaloosa, AL, USA, 2018.
- [85] Gao, J.W.; Xie, M.X.; Xia, M.X. Effects of boron and lanthanum on the impurity iron in aluminium melts. Miner. Process. Extr. Metall.: Trans. Inst. Min. Metall. 2011, 120, 172–176.
- [86] Hatch, J.E. Aluminium: Properties and Physical Metallurgy, 1st ed.; ASM International: Metals Park, OH, USA, 1984; pp. 1–397.
- [87] Bishara, H.; Lee, S.; Brink, T.; Ghidelli, M.; Dehm, G. Understanding grain boundary electrical resistivity in Cu: The effect of boundary structure. ACS Nano 2021, 15, 15397–16946.
- [88] Subedi, K.N.; Kappagantula, K.; Kraft, F.; Nittala, A.; Drabold, D.A. Electrical conduction processes in aluminum: Defects and Phonons. Phys. Rev. B 2022, 105, 104114.
- [89] Samuel, E.; Tahiri, H.; Samuel, A.M.; Samuel, F.H. Heterogenous grain nucleation in Al-Si alloys: Types of nucleant inoculation. Metals 2024, 14, 271.
- [90] Park, S.B. Heterogeneous nucleation models to predict grain size in solidification. Prog. Mater. Sci. 2022, 123, 100822.
- [91] Burke, J.E.; Turnbull, D. Recrystallization and grain growth. Prog. Phys. Met. 1952, 3, 220–244.
- [92] Chen, Q.; Chen, R.; Su, J.; He, Q.; Tan, B.; Xu, C.; Huang, X.; Dai, Q.; Lu, J. The mechanisms of grain growth of Mg Alloys: A review. J. Magnes. Alloy. 2022, 10, 2384–2397.
- [93] Stange, H.; Brunken, S.; Greiner, D.; Heinemann, M.D.; Kaufmann, C.A.; Schmidt, S.S.; Bäcker, J.P.; Klaus, M.; Genzel, C.; Mainz, R. Diffusion-induced grain boundary migration as mechanism for grain growth and defect annihilation in chalcopyrite thin films. Acta Mater. 2016, 111, 377–384.
- [94] Tavakkoli, V.; Boltynjuk, E.; Scherer, T.; Mazilkin, A.; Ivanisenko, Y.; Ungar, T.; Kübel, C. Precipitate-mediated enhancement of mechanical and electrical properties in HPTE-processed Al-Mg-Si alloy. Mater. Sci. Eng. A 2024, 906, 146556.
- [95] Gaber, A.; Afify, N.; El-Halawany, S.M.; Mossad, A. Studies on Al-Mg solid solutions using electrical resistivity and microhardness measurements. EPJ Appl. Phys. 1999, 7, 103–109.
- [96] Hou, J.P.; Wang, Q.; Zhang, Z.J.; Tian, Y.Z.; Wu, X.M.; Yang, H.J.; Li, X.W.; Zhang, Z.F. Nano-scale precipitates: The key to high strength and high conductivity in al alloy wire. Mater. Des. 2017, 132, 148–157.
- [97] Vončina, M.; Paulin, I.; Medved, J.; Petrič, M. Predicting the quality of grain refiners from electrical resistance measurements of aluminum. Metals 2023, 13, 717.
- [98] Murashkin, M.; Medvedev, A.; Kazykhanov, V.; Krokhin, A.; Raab, G.; Enikeev, N.; Valiev, R.Z. Enhanced mechanical properties and electrical conductivity in ultrafine-grained Al 6101 alloy processed via ECAP-conform. Metals 2015, 5, 2148–2164.
- [99] Yang, Z.; Mallow, S.; Banhart, J.; Kessler, O. Probing precipitation in aluminium alloys during linear cooling via in-situ differential scanning calorimetry and electrical resistivity measurement. Thermochim. Acta 2024, 739, 179815.
- [100] Eivani, A.; Ahmed, H.; Zhou, J.; Duszczyk, J. Correlation between electrical resistivity, particle dissolution, precipitation of dispersoids, and recrystallization behavior of AA7020 aluminum alloy. Metall. Mater. Trans. A 2009, 40, 2435–2446.
- [101] Wang, M.; Wu, J.; Yang, S.; Knezevic, M.; Huang, Z.; Zhao, Y.; Liu, T.; Shen, B.; Wang, J. Processing of an as-cast Al-7.5 wt%Y eutectic alloy by rolling and annealing to improve the tradeoff between strength and electrical conductivity. Mater. Sci. Eng. A 2024, 890, 145950.
- [102] Vandersluis, E.; Ravindran, C.; Bamberger, M. Mechanisms affecting hardness and electrical conductivity in artificially-aged B319 aluminum alloy. J. Alloys Compd. 2021, 867, 159121.
- [103] Li, Y.F.; Qin, J.; Yu, J.M.; Liu, Y.; Li, Z.; Nagaumi, H.; Zhang, B. A novel approach to improve conductivity of 1XXX Al alloy by in situ synthesis of Al-X-B grain refiner. Metall. Mater. Trans. A 2024, 55, 1707–1712.
- [104] Belov, N. Multicomponent Phase diagrams: Applications for Commercial Aluminum Alloys; Elsevier: Amsterdam, The Netherlands, 2005; pp. 1-413.
- [105] Sauvage, X.; Bobruk, E.V.; Murashkin, Y.M.; Nasedkina, Y.; Enikeev, N.A.; Valiev, R.Z. Optimization of electrical conductivity and strength combination by structure design at the nanoscale in Al-Mg-Si alloys. Acta Mater. 2015, 98, 355–366.
- [106] De Souza, D.F.; Salotti, F.A.M.; Sauer, I.L.; Tatizawa, H.; De Almeida, A.T.; Kanashiro, A.G. A performance evaluation of three-phase induction electric motors between 1945 and 2020. Energies 2022, 15, 2002.
9. 著作権:
この資料は、ユスフ・ゼイベック、セミーレ・カユシュ、エゲ・アヌル・ディレルの論文:「市販純アルミニウムの電気伝導率の向上:AlB8マスター合金と熱処理の相乗効果」に基づいています。
論文ソース: https://doi.org/10.3390/ma18020364
この資料は上記の論文に基づいて要約されたものであり、商業目的での無断使用は禁止されています。
Copyright © 2025 CASTMAN. All rights reserved.