本紹介論文は、「Journal of Manufacturing and Materials Processing (MDPI)」により発行された論文「[The Mechanical Properties of a Transient Liquid Phase Diffusion Bonded SSM-ADC12 Aluminum Alloy with a ZnAl4Cu3 Zinc Alloy Interlayer]」に基づいています。
![Table 2. The chemical compositions and mechanical properties of the SSM-ADC12 aluminum alloy and the ZnAl4Cu3 zinc alloy chosen for this study [18,19].](https://castman.co.kr/wp-content/uploads/image-2255-1024x289.webp)
1. 概要:
- 論文名: ZnAl4Cu3亜鉛合金中間層を用いた過渡液相拡散接合SSM-ADC12アルミニウム合金の機械的特性 (The Mechanical Properties of a Transient Liquid Phase Diffusion Bonded SSM-ADC12 Aluminum Alloy with a ZnAl4Cu3 Zinc Alloy Interlayer)
- 著者: Chaiyoot Meengam, Yongyuth Dunyakul and Dech Maunkhaw
- 発行年: 2024
- 発行学術誌/学会: Journal of Manufacturing and Materials Processing (J. Manuf. Mater. Process.)
- キーワード: transient liquid phase diffusion bonding; SSM-ADC12 aluminum alloy; semi-solid status; ZnAl4Cu3 Zinc alloy; interlayer materials
2. アブストラクト:
本研究では、半固体状態で行われる溶接プロセスである過渡液相拡散接合(TLPDB)後、ZnAl4Cu3亜鉛合金中間層を有するSSM-ADC12アルミニウム合金試験片の機械的特性を観察した。実験の目的は、接合温度(400、430、460、490、および520 °C)、接合時間(60、90、および120分)、およびZnAl4Cu3亜鉛合金の厚さ(0.5、1.0、および2.0 mm)といったパラメータが、機械的特性および形成される欠陥の種類にどのように影響するかを調査することであった。結果は、TLPDBプロセス後の異なるパラメータによって接合強度が著しく変化することを示している。最大接合強度32.21 MPaは、接合温度490 °C、接合時間20分、および厚さ2.0 mmのZnAl4Cu3亜鉛合金層で達成された。逆に、溶接パラメータを変更すると接合強度に影響を与えた。最小接合強度2.73 MPaは、接合温度400 °C、接合時間90分、および厚さ2.0 mmのZnAl4Cu3亜鉛合金中間層で達成された。ビッカース微小硬さの結果は、接合ゾーンがSSM-ADC12アルミニウム合金の母材(BMs)(86.60 HV)およびZnAl4Cu3亜鉛合金(129.37 HV)と比較して低い硬さ値を有することを示した。最大硬度は83.27 HVであり、これは接合温度520 °C、接合時間90分、および厚さ2.0 mmのZnAl4Cu3亜鉛合金から得られた。しかし、界面近傍では、MgZn2金属間化合物(IMCs)の形成により硬さ値が増加した。疲労結果は、SSM-ADC12アルミニウム合金のBMsにおける応力振幅が31.21 MPaであり、このTLPDBプロセスから得られる材料(TLPDB Material)における応力振幅が20.92 MPaであることを示した(繰り返し荷重の限界が10^6サイクルを超えた場合)。微細構造検査により、β共晶Si IMC再結晶構造からη(Zn–Al–Cu)およびβ(Al2Mg3Zn3) IMCsへの変態が明らかになった。SEMにより、幅6–11 µmおよび長さ16–44 µmへのサイズ縮小が観察された。最後に、この実験ではボイドまたは気孔および座屈欠陥が見つかった。
3. 緒言:
ADC12アルミニウム合金は、その軽量性と強度により、エンジン部品、トランスミッションケース、ホイール、構造部品などの自動車部品に一般的に使用されている[1]。一方、航空宇宙産業では、軽量で強度と耐熱性に優れた材料が要求される特定の航空宇宙部品にこれらの合金を適用している[2]。さらに、エレクトロニクス産業では、その熱伝導性と機械加工性により、電子機器のハウジング、ヒートシンク、コネクタなどの電子・電気部品にこれらの合金を適用している[3]。したがって、ADC12アルミニウム合金は、優れた鋳造性、比較的高い強度、良好な耐食性、良好な熱伝導性、および良好な機械加工性により、その使用率が継続的に増加している[4]。
ガス誘起半固体(GISS)プロセッシングは、特にアルミニウムベースの合金において、金属マトリックス複合材料および先端材料の製造に使用される方法である。この技術は、溶融金属マトリックスにガス気泡を導入し、球状微細構造を持つ半固体状態への遷移を引き起こすものである[5]。
ADC12アルミニウム合金は、一般的にGISSプロセスを用いて成形される。Janudomら[6]は、ADC12アルミニウム合金の半固体ダイカストの実現可能性を調査し、GISSプロセスを用いてこの種の材料を成形する大きな可能性を見出した。これにより、特性が向上し、微細構造の均一性が増した材料を製造できる。さらに、Gautam[7]は、ADC12 Al合金の半固体レオキャストプロセスを研究した。結果は、微細構造がほぼ球状を示し、マトリックス構造内で比較的均一に分布し、最良の機械的特性は引張強度223 MPa、伸び6.5%、硬さ87 VHであったことを示した。その結果、ADC12アルミニウム合金は、その優れた機械的特性により、半固体鋳造に適している。
過渡液相拡散接合(TLPDB)は、従来の溶接技術では接合できない金属材料を接合するために使用される特殊な溶接技術であり、特に高品質な接合を目指す航空宇宙産業で用いられる。このプロセスは、材料を密着させるために熱と圧力を加え、界面で拡散が起こるようにするものである。TLPDBの際立った特徴は、通常、中間層材料の添加による一時的な液相の導入である[8]。TLPDBは、融点や冶金学的特性の違いにより従来の方法では溶接が困難な場合がある、類似または異種の組成を持つ材料を接合するために一般的に使用される。中間層材料は、母材(BMs)よりも融点が低い材料の薄層である。これは接合される部品の間に配置される。この中間層は、接合プロセス中に一時的な液相として機能する。TLPDBメカニズムは、中間層の融点以上であるがBMsの融点以下の温度を使用する。これにより、BMsを固体のまま保ちながら中間層が溶融する。BMsからの原子が液相に拡散し、界面を横切る原子混合と接合を促進する。この拡散プロセスは、ボイドや欠陥を接合界面から排除し、強力な冶金学的接合をもたらすのに役立つ。同時に、接合プロセス中に材料に圧力が加えられ、接合面間の密着を確保し、界面を横切る原子の拡散を促進する[9,10]。TLPDBは、異種材料の接合能力を含む、従来の溶接技術に比べていくつかの利点を提供する。TLPDBは、組成や特性に大きな違いがある材料を接合するために使用でき、溶接が可能な用途の範囲を広げる。このプロセスは、BMsの融点よりも低い温度を使用するため、歪み、熱影響部(HAZ)形成、および高い接合強度を持つBMsの冶金学的変化のリスクを最小限に抑える。これは、接合プロセス中の界面を横切る原子の拡散が、優れた機械的特性を持つ強力な冶金学的接合をもたらすためである。最後に、接合界面の微細構造と組成の精密な制御により、溶接継手の性能と信頼性が向上する[11]。TLPDBパラメータが機械的特性に著しく影響することは明らかである。したがって、TLPDBのパラメータ選択は重要であり、特に微細構造における接着と金属間化合物(IMCs)の形成に重要な役割を果たすセメント質材料の選択が重要である。研究されてきた重要なTLPDBパラメータをTable 1にまとめる。
4. 研究の概要:
研究テーマの背景:
ADC12アルミニウム合金は、望ましい微細構造を達成するためにしばしばGISS技術を用いて処理され、様々な産業で不可欠である。TLPDBは、特に高品質な接合を目指し、異種材料や従来の方法では溶接が困難な材料を接合する場合に、このような材料に適した高度な接合技術である。中間層材料の選択を含むTLPDBプロセスのパラメータは、接合部の機械的特性と微細構造に著しく影響する。
先行研究の状況:
これまでの研究では、様々なアルミニウム合金やその他の材料についてTLPDBが調査されており、異なる中間層材料やプロセスパラメータが検討されてきた(論文のTable 1に要約)。例えば、Al2219とCu中間層[12]、Al6063と銅箔[13]、Al-Mg-Si合金とSn系材料[14]、AR500鋼とAA7075とAl-Si-Zn中間層[15]、1420 Al-Li合金[16]についてTLPDBが研究されてきた。しかし、GISS技術を用いたSSM-ADC12アルミニウム合金とZnAl4Cu3亜鉛合金中間層の特定の組み合わせ、および接合温度、時間、中間層厚さの影響に関する詳細な調査は、新たな研究分野である。
研究の目的:
本実験の目的は、以下のTLPDBパラメータ、すなわち接合温度(400、430、460、490、および520 °C)、接合時間(60、90、および120分)、およびZnAl4Cu3亜鉛合金中間層の厚さ(0.5、1.0、および2.0 mm)が、SSM-ADC12アルミニウム合金接合部の機械的特性(接合強度、疲労、硬さ)および形成される欠陥の種類にどのように影響するかを調査することであった。本研究はまた、IMC相変態のメカニズムを評価することも目的とした。
研究の核心:
本研究の核心は、ZnAl4Cu3亜鉛合金を中間層材料として用いてSSM-ADC12アルミニウム合金試験片にTLPDBを実施することであった。実験では、接合温度、接合時間、および中間層の厚さを系統的に変化させた。得られた接合部は、その後、光学顕微鏡、SEM、EDXなどの技術を用いて、その機械的特性(接合強度、疲労寿命、微小硬さ)および微細構造的特徴(IMC形成、欠陥の存在、相変態)について評価された。
5. 研究方法論
研究デザイン:
本研究は実験的研究として設計された。GISS技術を用いて形成されたSSM-ADC12アルミニウム合金を母材として使用した。ZnAl4Cu3亜鉛合金(市販グレードZA27)を中間層材料として用いた。TLPDBプロセスは、接合温度(400、430、460、490、および520 °C)、接合時間(60、90、および120分)、および中間層の厚さ(0.5、1.0、および2.0 mm)を、アルゴン雰囲気中で3.4 MPaの一定の接合圧力下で変化させて実施した。
データ収集と分析方法:
- Materials: GISS技術で形成されたSSM-ADC12アルミニウム合金(融点548 °C)およびZnAl4Cu3亜鉛合金中間層(融点399 °C)。化学組成および機械的特性はTable 2に詳述。
- TLPDB Process: 円筒形のSSM-ADC12試料(長さ45 mm、直径10 mm)およびZnAl4Cu3中間層ディスク(直径10 mm、厚さ0.5、1.0、2.0 mm)を使用した。表面は研磨および洗浄された。接合はアルゴンガスフロー(7 L/min)のあるガスチャンバー内で行われた。TLPDB中の温度制御はFigure 3に示す。
- Fatigue Testing: NARIN NRI-CPT500-2 静的および動的試験機を使用し、20 Hz、応力比R = −1で実施した。ストローク値は調整した(0.35~0.80 mm)。疲労限度は10^6サイクル以上とした。S–N曲線を生成した。試験片はASTM E466-15に従って準備した。
- Metallurgy and Mechanical Testing:
- 接合強度試験は、ASTM E8M-04に従い、Lloyd EZ50万能試験機(クロスヘッド速度1.67 × 10^−2 mm/s)を用いて室温で実施した。
- 微小硬さは、FM-700eビッカース微小硬さ試験機(荷重100 g、保持時間10 s)を用いて評価し、圧痕は接合中心から0.2 mmの位置に付けた。
- 微細構造検査は、切断、研磨(SiC紙P320-P1200、アルミナ粉末5.0、3.0、1.0 µm)、およびケラー試薬によるエッチングを含んだ。分析は、BH2-UMA光学顕微鏡および化学組成分析用のEDXを備えたFEI-Quanta 400電子顕微鏡(SEM)を用いて行った。
研究テーマと範囲:
本研究は、TLPDBパラメータ(接合温度、接合時間、中間層厚さ)が、SSM-ADC12アルミニウム合金とZnAl4Cu3亜鉛合金中間層との接合部の機械的特性(接合強度、疲労、硬さ)および微細構造進化(IMC相変態、欠陥形成)に及ぼす影響に焦点を当てた。本研究は、接合温度400~520 °C、接合時間60~120分、中間層厚さ0.5、1.0、および2.0 mmを対象とした。
6. 主な結果:
主な結果:
- Physical Characteristics: 接合時間と温度は試料の変形に影響を与えた。時間が長く、温度が高いほど、ZnAl4Cu3中間層の排出が多くなり、偏向欠陥の可能性が生じた (Figure 4)。
- Bonding Strength: 接合強度は温度、時間、中間層の厚さに依存した (Figure 5)。最大接合強度32.21 MPaは、490 °C、120分の接合時間、2.0 mmの中間層厚さで達成された。最小2.73 MPaは、400 °C、90分、2.0 mmの厚さで記録された。接合時間と温度の増加は、一般的に拡散とIMC形成の改善、および表面酸化膜の除去により接合強度を増加させた。Table 3はこれらの結果を先行研究と比較している。
- Fatigue Analysis: TLPDB材料は、SSM-ADC12 BM(10^6サイクルで31.12 MPa)と比較して低い疲労耐久限度(10^6サイクルで20.29 MPa)を示した (Table 4, Figure 6, Table 5)。これは、TLPDBプロセスからの新しい析出物(β-eutectic相、α-primaryアルミニウムマトリックス相)および亀裂/ボイド欠陥に起因する。
- Microstructure:
- 適切な接合温度と時間によりボイドは消滅した。微細なMgZn2粒子が形成され、α-Al相中に分散した。Znの急速な拡散によりβ + η相が形成され、β(Al2Mg3Zn3)はβ'に過飽和し、η(Zn-Al–Cu)はβに合体してη'を形成した。ZnAl4Cu3亜鉛合金の収縮は、高温(520 °C)での急冷により接合ゾーンに亀裂を生じさせた (Figure 7a-c)。
- 490 °C、90分、2.0 mm中間層では、欠陥の少ない完全な拡散が観察された (Figure 7d-f)。
- BMs中の共晶Si IMCsは、TLPDB後、球状から歪んだ粒子に変形し、粒子サイズは78–97 µmから118–139 µmに増加した (Figure 7g)。
- SEM分析 (Figure 8) は、共晶Si IMCsの変形を示した。BMsでは、それらはガーゼ状であった(長さ21–70 µm、幅2–9 µm)。接合ゾーン、HAZ、NBZでは、Si IMCsはより小さく細長くなった(例:HAZでは長さ6–11 µm、幅2–4 µm、NBZでは長さ16–44 µm、幅2–3 µm)。中央中間層では、Si IMCsは微細な粒子サイズを有していた(長さ12–27 µm、幅19–29 µm)。
- EDXマッピング (Figure 9, Table 6) は、接合ゾーンにAl (65.25 wt%)、Zn (19.01 wt%)、C (9.94 wt%)、Si (5.68 wt%)、およびCu (2.34 wt%) が存在することを示した。
- Vickers Microhardness: 接合ゾーンの硬さは、IMC析出(特にMgZn2)により高温で上昇した。最大硬度83.20 HVは、520 °C、120分の接合時間、2.0 mm中間層で達成された (Figure 10c)。不十分な接合時間/温度は低い硬度をもたらした (Figure 10a,b)。η(Zn-Al–Cu)、β(Al2Mg3Zn3)、およびMgZn2 IMCsが硬度増加に寄与した。
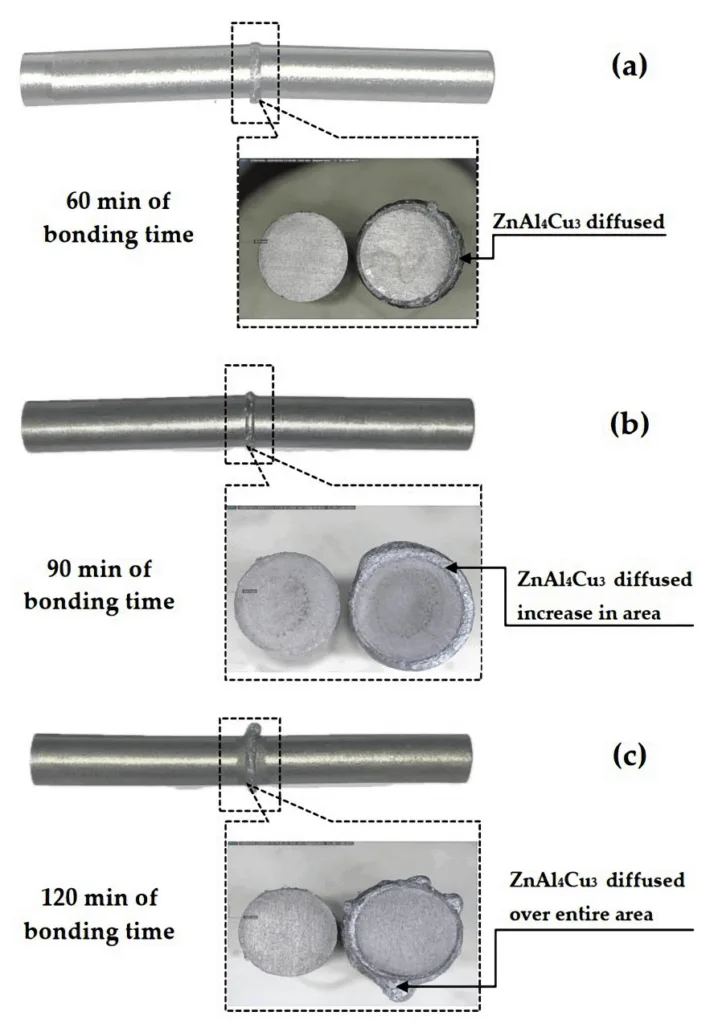
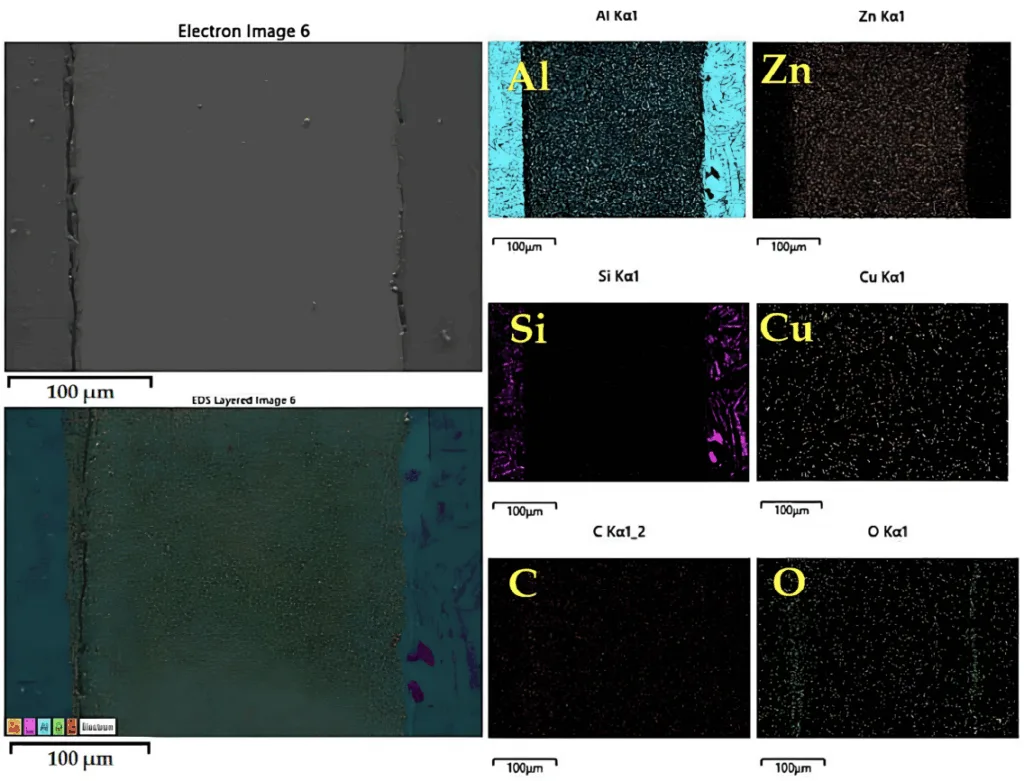
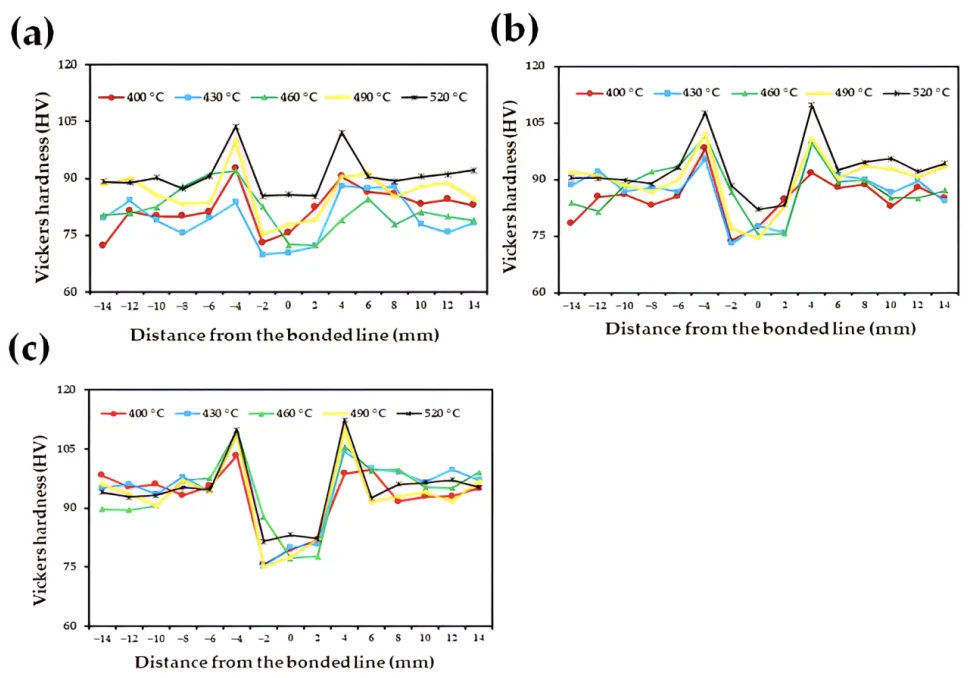
図のリスト:
- Figure 1. Photograph of the base microstructure of the SSM-ADC12 aluminum alloy formed using GISS casting.
- Figure 2. Equipment used for the TLPDB of SSM-ADC12 aluminum alloy using the ZnAl4Cu3 zinc alloy interlayer material.
- Figure 3. A schematic view of the temperature of TLPDB of the SSM-ADC12 aluminum alloy.
- Figure 4. Micrographs showing the characteristics of the samples and cross sections after TLPDB of the SSM-ADC12 aluminum alloy using a ZnAl4Cu3 zinc alloy with a 2.0 mm interlayer at 490 °C for (a) 60 min, (b) 90 min, and (c) 120 min.
- Figure 5. Bond strengths for the TLPDB of the SSM-ADC12 aluminum alloy using a ZnAl4Cu3 zinc alloy as the interlayer with different bonding times of (a) 60 min, (b) 90 min, and (c) 120 min.
- Figure 6. The fatigue S-N curves for the BMs and TLPDB material of the SSM-ADC12 aluminum alloy using a ZnAl4Cu3 zinc alloy as an interlayer.
- Figure 7. Micrographs of the microstructure in the bonded zone (a-g) and BM around diffused area after TLPDB.
- Figure 8. SEM micrographs (taken in EDX mode) of different characteristics of the eutectic Si IMCs show the following: (a) entire bond, (b) BMs, (c) bonding zone, (d) near-bonding zone, and (e) center interlayer.
- Figure 9. EDX mapping analysis of the composition (wt%) in the bonded zone.
- Figure 10. The Vickers microhardness values of the SSM-ADC12 aluminum alloy after TLPDB with a ZnAl4Cu3 zinc alloy interlayer that was 2.0 mm thick: (a) 60 min bonding time, (b) 90 min bonding time, and (c) 120 min bonding time.
7. 結論:
本研究は、ZnAl4Cu3亜鉛合金中間層を用いたSSM-ADC12アルミニウム合金接合部に対するTLPDBパラメータの影響を成功裏に調査した。主な結論は以下の通りである。
- 平均最大接合強度32.21 MPaは、接合温度490 °C、接合時間120分、およびZnAl4Cu3亜鉛合金中間層材料厚さ2.0 mmで得られた。
- TLPDB材料の評価後、亀裂、ボイド、および変形欠陥が検出された。
- TLPDB材料およびBMsの耐久限度は、それぞれ20.29 MPaおよび31.12 MPaであった。
- 最大ビッカース微小硬さ83.20 HVは、120分の接合、520 °Cの接合温度、および厚さ2.0 mmのZnAl4Cu3亜鉛合金中間層で得られた。一方、η(Zn-Al–Cu)、β(Al2Mg3Zn3)、およびMgZn2金属間化合物(IMCs)が硬度の上昇をもたらした。
- 接合線近傍でのMgZn2相の形成と析出は、機械的特性の向上をもたらした。β共晶Si IMCsを有するα初晶マトリックスからη(Zn-Al–Cu)相への変態も接合線で観察された。光学顕微鏡による評価では、析出物が球状から粗大な構造へと変化し、粒子が大きくなることが示された。一方、SEM評価では、β共晶Si IMCsがβ(Al2Mg3Zn3)およびMgZn2 IMCsへと拡散し、平均幅19–29 µm、平均長さ12–27 µmとなることが示された。最後に、接合部でのEDXマッピングにより、Mg、Si、およびAlが自由に移動できることが示された。
8. 参考文献:
- [1] Lee, H.; Yoon, J.; Yoo, J. Manufacturing of Aerospace Parts with Diffusion Bonding Technology. Appl. Mech. Mater. 2011, 87, 182–185.
- [2] Pola, A.; Tocci, M.; Kapranos, P. Microstructure and Properties of Semi-Solid Aluminum Alloys: A Literature Review. Metals 2018, 8, 181.
- [3] Vinith, S.; Uthayakumar, A.; Rajan, S. Fluidity of ADC12 alloy based on theoretical and computational fluid dynamics. Mech. Eng. 2015, 4, 996–999.
- [4] JIS H 5302:Japan; Japanese Industrial Standard, Aluminum Alloys Die Castings (ADC 12). Japanese Industrial Standard: Tokyo, Japan, 2000; p. 10.
- [5] Wannasin, J.; Janudom, S.; Rattanochaikul, T.; Canyook, R.; Burapa, R.; Chucheep, T.; Thanabumrungkul, S. Research and development of gas induced semi-solid process for industrial applications. Trans. Nonferr. Met. Soc. China 2010, 20, 1010–1015.
- [6] Janudom, S.; Rattanochaikul, T.; Burapa, R.; Wisutmethangoon, S.; Wannasin, J. Feasibility of semi-solid die casting of ADC12 aluminum alloy. Trans. Nonferr. Met. Soc. China 2010, 20, 1756–1762.
- [7] Gautam, S.K.; Singh, B.K. Investigation on the effects of isothermal holding temperature and time on the coarsening mechanism and rheological properties of ADC12 Al semi-solid slurry. Mater Chem Phys. 2024, 314, 128813.
- [8] Simões, S. Diffusion Bonding and Brazing of Advanced Materials. Metals 2018, 8, 959.
- [9] Zhang, L.X.; Chang, Q.; Sun, Z.; Xue, Q.; Feng, J.C. Effects of boron and silicon on microstructural evolution and mechanical properties of transient liquid phase bonded GH3039/IC10 joints. J. Manuf. Process. 2019, 38, 167–173.
- [10] Jiao, Y.J.; Sheng, G.M.; Zhang, Y.T.; Xu, C.; Yuan, X.J. Transient liquid phase bonding of Inconel 625 with Mar-M247 superalloy using Ni-Cr-B interlayer: Microstructure and mechanical properties. Mater. Sci. Eng. A 2022, 831, 142204.
- [11] Yuan, L.; Ren, J.; Xiong, J.T.; Zhao, W.; Shi, J.M.; Li, J.L. Transient liquid phase bonding of Ni3Al based superalloy using Mn-Ni-Cr filler. J. Mater. Res. Technol. 2021, 11, 1583–1593.
- [12] Vatnalmath, M.; Auradi, V.; Murthy, B.V.; Nagaral, M.; Pandian, A.A.; Islam, S.; Khan, M.S.; Anjinappa, C.; Razak, A. Impact of Bonding Temperature on Microstructure, Mechanical, and Fracture Behaviors of TLP Bonded Joints of Al2219 with a Cu Interlayer. ACS Omega 2023, 8, 26332–26339.
- [13] Saleh, M.I.; Roven, H.J.; Khan, T.I.; Iveland, T. Transient Liquid Phase Bonding of Al-6063 to Steel Alloy UNSS32304. J. Manuf. Mater. Process. 2018, 2, 58.
- [14] Dong, J.H.; Liu, H.; Ji, S.D.; Yan, D.J.; Zhao, H.X. Diffusion Bonding of Al-Mg-Si Alloy and 301L Stainless Steel by Friction Stir Lap Welding Using a Zn Interlaye. Materials 2022, 15, 696.
- [15] Muhamed, M.N.; Omar, M.Z.; Abdullah, S.; Sajuri, Z.; Zamri, W.F.H.W. Al-Si-Zn Behaviouron Interface of AR500 Steel and AA7075 Aluminium Alloy BrazedJoint. J. Phys. Conf. Ser. 2020, 1532, 012006.
- [16] Zhang, H.; Niu, T.; Yuan, M. Influence of High Magnetic Field-Thermal Coupling Processing on Diffusion Bonding Properties and Element Diffusion of 1420 Al-Li Alloy. Crystals 2022, 12, 1508.
- [17] Canyook, R.; Wannasin, J.; Wisutmethangoon, S.; Flemings, M.C. Characterization of the microstructure evolution of a semi-solid metal slurry during the early stages. Acta Mater. 2012, 60, 3501–3510.
- [18] Chainarong, S.; Pitakaso, R.; Sirirak, W.; Srichok, T.; Khonjun, S.; Sethanan, K.; Sangthean, T. Multi-Objective Variable Neighborhood Strategy Adaptive Search for Tuning Optimal Parameters of SSM-ADC12 Aluminum Friction Stir Welding. J. Manuf. Mater. Process. 2021, 5, 123.
- [19] Seah, K.H.W.; Sharma, S.C.; Girish, B.M. Mechanical properties of cast ZA-27 graphite particulate composites. Mater. Des. 1996, 16, 271–275.
- [20] Kittima, S.; Yoshiharu, M.; Yukio, M.; Nobushiro, S. Fatigue Strength Estimation Based on Local Mechanical Properties for Aluminum Alloy FSW Joints. Metals 2017, 10, 186.
- [21] ASTM E466-15; Standard Practice for Conducting Force Controlled Constant Amplitude Axial Fatigue Tests of Metallic Materials. Designation. ASTM International Standards: West Conshohocken, PA, USA, 2015.
- [22] ASTM E8; Standard Test Methods for Tension Testing of Metallic Materials. Designation. ASTM International Standards: West Conshohocken, PA, USA, 2015.
- [23] Malekan, A.; Farvizi, M.; Mirsalehi, S.E.; Saito, N.; Nakashima, K. Holding Time Influence on Creep Behavior of Transient Liquid Phase Bonded Joints of Hastelloy X. Mater. Sci. Eng. A 2020, 772, 138694.
- [24] Al Hazaa, A.; Haneklaus, N.; Almutairi, Z. Impulse pressure-assisted diffusion bonding (IPADB): Review and outlook. Metals 2021, 11, 323.
- [25] Venugopal, S.; Seeman, M.; Seetharaman, R.; Jayaseelan, V. The effect of bonding process parameters on the microstructure and mechanical properties of AA5083 diffusion-bonded joints. Int. J. Ligh. Mater. Manuf. 2022, 5, 555–563.
- [26] Yu, W.; Zhao, H.; Huang, Z.; Chen, X.; Aman, Y.; Li, S.; Zhai, H.; Guo, Z.; Xiong, S. Microstructure evolution and bonding mechanism of Ti2SnC–Ti6Al4V joint by using Cu pure foil interlayer. Mater. Charact. 2017, 127, 53–55.
- [27] Silva, M.; Ramos, A.; Vieira, M.; Simões, S. Diffusion Bonding of Ti6Al4V to Al2O3 Using Ni/Ti Reactive Multilayers. Metals 2021, 11, 655.
- [28] Ben-Haroush, M.; Mittelman, B.; Priel, R.S.E. The Influence of Time, Atmosphere and Surface Roughness on the Interface Strength and Microstructure of AA6061–AA1050 Diffusion Bonded Components. Materials 2023, 16, 769.
- [29] Meengam, C.; Dunyakul, Y.; Maunkhaw, D.; Chainarong, S. Transient Liquid Phase Bonding of Semi-Solid Metal 7075 Aluminum Alloy Using ZA27 Zinc Alloy Interlayer. Metals 2018, 8, 637.
- [30] Alhazaa, A.; Khan, T.; ul Haq, I. Transient liquid phase (TLP) bonding of Al7075 to Ti–6Al–4V alloy. Mater. Charact. 2010, 61, 312–317.
- [31] Wen, Z.; Li, Q.; Liu, F.; Dong, Y.; Zhang, Y.; Hu, W.; Li, L.; Gao, H. Transient Liquid Phase Diffusion Bonding of Ni3Al Superalloy with Low-Boron Nickel-Base Powder Interlayer. Materials 2023, 16, 2554.
- [32] Kejanli, H.; Taşkin, M.; Kolukisa, S.; Topuz, P. Transient liquid phase (TPL) diffusion bonding of Ti45Ni49Cu6 P/M components using Cu interlayer. Int. J. Adv. Manuf. Technol. 2009, 44, 695–699.
- [33] Meengam, C.; Dunyakul, Y.; Chainarong, S.; Maunkhaw, D. The Influence of Diffuse Element in Solid-State on Dissimilar Joint between Semi-Solid Cast 7075 with 6061 Al Alloy by Diffusion Welding. Solid State Phenom. 2022, 330, 71–76.
- [34] Maity, J.; Pal, T.K.; Maiti, R. Transient liquid phase diffusion bonding of 6061-15 wt % SiCp in argon environment. J. Mater. Process. Technol. 2009, 209, 3568–3580.
- [35] Seyyed Afghahi, S.S.; Ekrami, A.; Farahany, S.; Jahangiri, A. Fatigue properties of temperature gradient transient liquid phase diffusion bonded Al7075-T6 alloy. Trans. Nonferrous Met. Soc. China 2015, 25, 1073–1079.
- [36] Liu, Y.; Zhang, Y.; Liu, S.; Xiao, S.; Sun, Y.; Wang, X. Effect of unbonded areas around hole on the fatigue crack growth life of diffusion bonded titanium alloy laminates. Eng. Fract. Mech. 2016, 1, 176–188.
- [37] He, X.; Liu, W.; Sun, Y.; Wang, X. Fatigue crack growth characteristic for diffusion bonded laminates of titanium alloy with centered hole. Eng. Mech. 2015, 4, 244–249.
- [38] Syed, A.K.; Zhang, X.; Moffatt, J.E.; Maziarz, R.; Castelletti, L.; Fitzpatrick, M.E. Fatigue performance of bonded crack retarders in the presence of cold worked holes and interference-fit fasteners. Int. J. Fatigue 2017, 105, 111–118.
- [39] Liu, Y.; Liu, S. Experimental Research on Fatigue Crack Growth Behavior of Diffusion-Bonded Titanium Alloy Laminates with Preset Unbonded Areas. Materials 2022, 15, 5224.
- [40] Li, S.; Xuan, F.; Tu, S. Fatigue damage of stainless steel diffusion-bonded joints. Mater. Sci. Eng. A 2008, 480, 125–129.
- [41] Abdolvand, R.; Atapour, M.; Shamanian, M.; Allafchian, A. The effect of bonding time on the microstructure and mechanical properties of transient liquid phase bonding between SAF 2507. J. Manuf. Process. 2017, 25, 172–180.
- [42] Norouzi, E.; Atapour, M.; Shamanian, M.; Allafchian, A. Effect of bonding temperature on the microstructure and mechanical properties of Ti-6Al-4V to AISI 304 transient liquid phase bonded joint. Mater. Des. 2016, 99, 543–551.
- [43] Habisch, S.; Peter, S.; Grund, T.; Mayr, P. The Effect of Interlayer Materials on the Joint Properties of Diffusion-Bonded Aluminium and Magnesium. Metals 2018, 8, 138.
- [44] Yang, L.; Yang, Y.; Zhang, Y.; Xu, F.; Jian, Q.; Lu, W. Microstructure evolution and mechanical properties of the In-Sn-20Cu composite particles TLP bonding solder joints. Appl. Phys. A 2020, 126, 343.
- [45] Kim, S.H.; Cha, J.-H.; Jang, C.; Sah, I. Microstructure and Tensile Properties of Diffusion Bonded Austenitic Fe-Base Alloys—Before and After Exposure to High Temperature Supercritical-CO2. Metals 2020, 10, 480.
- [46] Ghaderi, S.; Karimzadeh, F.; Ashra, A. Evaluation of microstructure and mechanical properties of transient liquid phase bonding of Inconel 718 and nano/ultrafine-grained 304L stainless steel. J. Manuf. Process. 2020, 49, 162–174.
- [47] Peng, Y.Y.; Li, C.; Li, H.J.; Liu, Y.C. Effect of interlayer on microstructure and mechanical properties of diffusional-bonded Ni3Al-based superalloy/S31042 steel joint. J. Manuf. Process. 2021, 72, 252–261.
9. 著作権:
- 本資料は、「Chaiyoot Meengam, Yongyuth Dunyakul and Dech Maunkhaw」による論文です。「The Mechanical Properties of a Transient Liquid Phase Diffusion Bonded SSM-ADC12 Aluminum Alloy with a ZnAl4Cu3 Zinc Alloy Interlayer」に基づいています。
- 論文の出典: https://doi.org/10.3390/jmmp8050184
本資料は上記論文に基づいて要約したものであり、商業目的での無断使用を禁じます。
Copyright © 2025 CASTMAN. All rights reserved.